Proceedings of the 29th Meeting
Working Group on Prolamin Analysis
and Toxicity (PWG)
Edited by Peter Koehler
German Research Centre for Food Chemistry, Freising
Verlag Deutsche Forschungsanstalt für Lebensmittelchemie - 2016
Preface
The Austrian City of Tulln was the venue of the 29th meeting of the Working Group on Prolamin Analysis and Toxicity from 8 to 10 October 2015. The meeting was hosted
by Romer Labs Division Holding GmbH, and the Austrian Coeliac Society assisted in
the registration of the participants. Simone Schreiter, the local organiser, was present
during the entire meeting. More than 60 persons participated in the meeting. This
shows the ongoing scientific significance of the topic gluten and gluten
hypersensitivities. From the 12 current members of the PWG, nine participated in the
meeting. Peter Koehler, chairman of the PWG, welcomed the group, one invited
speaker, participants from industry, research institutes as well as delegates from
European coeliac associations. Industry delegates came from starch producers,
manufacturers of gluten-free foods, and producers of analytical test kits for gluten
quantitation.
Analytical and clinical work in the field of coeliac disease and gluten done in the labs
of the PWG members as well as results of guest speakers were presented in 15 talks
and intensely discussed at the meeting. In addition, two presentations addressed
regulatory aspects of gluten analysis and labelling. A symposium on “Innate Immunity
and Coeliac Disease” with three presentations of internationally recognized experts
highlighted the latest advances in the field of the early steps of CD pathogenesis and
non-celiac gluten/wheat sensitivity.
At this occasion, I would like to express my thanks to all participants for their active
contributions and to all persons that made the meeting possible. I would like to thank
the local organizing team, in particular Simone Schreiter of Romer Labs and also
Christian Petz and Hertha Deutsch of the Austrian Coeliac Society, who perfectly
organized the hotel, the venue, and the registration. Also, very special thanks go to
Katharina Scherf for her help in proofreading. Finally, I would like to express my
appreciation to all friends, colleagues and sponsors for their ongoing support of the
PWG and the meeting.
Freising, March, 2016 Peter Koehler
1. Executive Summary
Among the topics of the meeting were analytical issues of gluten, novel approaches for
coeliac disease diagnosis, aspects of the innate and adaptive immune response, as well
as legal issues and standardization activities.
Analytical session
Six presentations were given in this session. The opening lecture and an additional talk
within the session discussed the requirements and current activities in the production
of suitable reference materials for gluten quantitation. Another analytical topic was the
specificity of different immunochemical kits for gluten quantitation towards gluten
fractions and gluten from different plant species. Two presentations were on the
detection and quantitation of gluten by mass spectrometry and, finally, breeding
approaches on reducing or elimination of coeliac disease activity of wheat and barley
were presented.
Clinical session
This session also included six presentations. The first talk was on the gluten-free diet
of the Spanish coeliac population and the nutrient intake compared to the glutencontaining
diet. Another presentation was on signalling in the innate immune response
in coeliac disease. A novel approach for endomysium antibody testing by using liver
tissue instead of oesophagus tissue was also presented. A talk on the use of mass
cytometry to image cell populations in different coeliac disease conditions was
followed by a presentation on the activity of avenin from oats in coeliac disease.
Symposium: Innate Immunity and Coeliac Disease
Three recognized experts in innate immunity presented results from their research.
Viral infections as well as gliadin peptides were discussed as potential triggers of the
innate response of the immune system in coeliac disease pathogenesis. Finally,
research on amylase-trypsin-inhibitors (ATI) was presented, which are thought to be
related to the innate response of the immune system. They have been postulated to act
as triggers of non-celiac gluten sensitivity but also as “second” and “third hits” in the
pathogenesis of a number of chronic inflammatory diseases.
4. Analytical research reports
4.1 Comparative analysis of prolamin and glutelin fractions with ELISA test kits
Barbara Lexhaller, Christine Tompos, Peter Koehler, Katharina A. Scherf
Deutsche Forschungsanstalt für Lebensmittelchemie, Leibniz Institut, Freising,
Germany
Introduction
Immunochemical methods such as enzyme-linked immunosorbent assays (ELISAs)
are specific and sensitive analytical tools for gluten quantitation. They do not require
specialised equipment, are comparatively fast and easy to use also in routine
applications and recommended by legislation [1]. Due to these advantages and lack of
a fully validated alternative method to quantitate gluten proteins, ELISAs are most
commonly used to monitor the safety of gluten-free foods for coeliac disease (CD)
patients. More than 20 ELISA test kits for gluten analysis are currently on the market.
Each of these test kits has its specific features regarding the procedure to extract gluten
proteins from the food matrix, the test format (sandwich or competitive), the reference
material used for calibration (PWG-gliadin [2], gluten or wheat protein), the type of
antibody (monoclonal or polyclonal), and the specificity and sensitivity of the antibody
against different epitopes depending on the antigen that was originally used for
immunisation (Tab. 1). In addition to various polyclonal antibodies (pAbs), current
sandwich ELISA test kits are based on the R5 [3], G12 [4], and 401.21 (Skerritt) [5]
monoclonal antibodies (mAbs). Gluten is composed of the alcohol-soluble prolamin
fraction and the alcohol-insoluble glutelin fraction that is only soluble after addition of
disaggregating and reducing agents. Analytical methods should be capable of detecting
the total gluten content, because both prolamins and glutelins harbour CDimmunogenic
epitopes [6]. Most ELISA test kits are assumed to only recognize the
prolamin fraction and the gluten content is calculated by multiplying the prolamin
content by a factor of two. However, detailed comparative studies on antibody
sensitivities and specificities against the prolamin and glutelin fractions from wheat,
rye, and barley are missing. Therefore, the aim of this study was to compare these
different gluten fractions within one ELISA test kit as well as to compare the same
gluten fraction between test kits.
Materials and methods
Modified Osborne fractionation and quantitation by RP-HPLC
Flours of wheat (cultivar Akteur), rye (cultivar Visello), and barley (cultivar Marthe)
were sequentially extracted with dilute salt solution (albumin/globulin fraction), 60% (v+v) aqueous ethanol (prolamin fraction) and 50% (v+v) 1-propanol/0.1 mol/L Tris-
HCl, pH 7.5 containing 0.06 mol/L (w+v) dithiothreitol at 60 °C under nitrogen
(glutelin fraction) [7]. After centrifugation, the respective extracts were made up to
volume (2 mL), filtered (0.45 μm) and the gluten protein concentrations were
quantitated by RP-HPLC using PWG-gliadin [2] as calibration reference.
Analysis with ELISA test kits
The fresh prolamin and glutelin extracts were diluted appropriately to fit within the
respective calibration range of the five sandwich ELISA test kits (Tab. 1).
Table 1. Overview of the differences in commercially available sandwich ELISA test
kits used for the comparative analysis of prolamin and glutelin fractions.
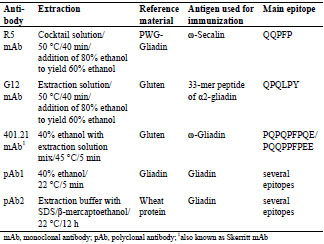
Five serial dilutions of each prolamin and glutelin extract were measured in duplicate
with each test kit. The last dilution step was always performed with the sample
dilution buffer included in the test kit and the ELISA procedures were carried out
strictly according to the manufacturers’ instructions.
Data analysis
The prolamin and glutelin concentrations quantitated by RP-HPLC were plotted
against the absorbance which was measured by the ELISA test kit. Then the ELISA
protein concentrations were calculated using the respective reference material provided as calibrator in the test kit. This allowed creating plots of the prolamin and
glutelin concentrations quantitated by ELISA against those quantitated by RP-HPLC
(Fig. 1). Ideally, both concentrations would be the same.
Results and discussion
Overall, the comparative analysis of prolamin and glutelin fractions from wheat, rye
and barley with five commercial ELISA test kits for gluten quantitation covered three
different mAbs (R5, G12 and Skerritt) as well as two pAbs. Taking the respective
dilutions into account, the prolamin and glutelin concentrations quantitated by RPHPLC
were always used as independent base values for all calculations and
comparisons of ELISA results.
The specificities and sensitivities of each antibody against the different gluten
fractions were variable within one test kit (Fig. 1). The R5 mAb had the highest
affinity towards rye and barley prolamins as well as rye glutelins. Wheat prolamins
were detected with about equal sensitivity as the kit standard, which was according to
expectations, because PWG-gliadin is used for calibration. The glutelins of wheat and
barley only showed very limited reactivity with the R5 mAb. Wheat, rye and barley
prolamins were detected by the G12 mAb with almost equal sensitivity that was also
comparable to the kit standard. However, the affinity of the G12 mAb towards wheat
and rye glutelins was very low and it was unable to detect barley glutelins, as has been
reported before [8]. In contrast to the R5 and G12 mAbs, the 401.21 (Skerritt) mAb
reacted with wheat, rye and barley glutelins with even higher sensitivity than with the
kit standard. Wheat and rye prolamins and the kit standard were detected with
comparable sensitivity, but the affinity towards barley prolamins was very low. Both
pAbs differed considerably in their abilities to accurately detect wheat, rye and barley
prolamins and glutelins. Wheat and rye prolamins were recognized by the pAb 1, but
all other fractions showed very limited reactivity. The pAb 2 detected all fractions with
high sensitivity except for barley glutelins.
When comparing the analyses of the same gluten fraction with the five different test
kits, the results also showed a high degree of variability. The only exception were
wheat prolamins (gliadins), which were only slightly overestimated by the R5, Skerritt
and pAb 2 assays and slightly underestimated by the G12 and pAb 1 assays. This
result was according to expectations, because most test kits are calibrated against
gliadins. However, wheat glutelins were overestimated about 9-fold by the Skerritt
mAb and underestimated by a factor of about 10 by the R5 and G12 mAbs and the
pAb 1, whereas the pAb 2 yielded results that agreed with the RP-HPLC quantitation.
In the case of rye prolamins, the R5 and pAb 2 assays overdetermined the
concentration, the pAb 1 assay slightly underestimated the concentration and the G12
and Skerritt mAb assays showed a good correlation to the RP-HPLC results. The
immunochemical determination of rye glutelins revealed that this fraction was
overestimated by the R5, Skerritt and pAb 2 assays, but underestimated by up to 10-
fold by the G12 and pAb 1 assays. The analyses of barley gluten fractions showed the highest overall degree of variability. Barley prolamins were overestimated by up to 6-
fold using the R5 and pAb 2 assays, but the pAb 1 and Skerritt assays hardly showed
any detection. The G12 assay resulted in quite accurate values. In contrast, the Skerritt
assay was the only one that was able to detect barley glutelins. All other mAbs and
pAbs showed virtually no affinity towards barley glutelins.
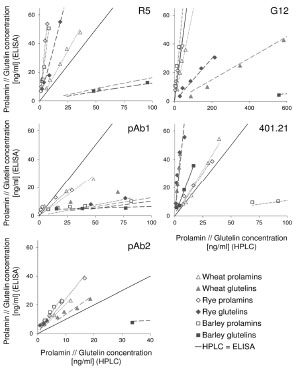
Figure 1. Prolamin and glutelin concentrations [ng/mL] quantitated by RP-HPLC
plotted against the prolamin and glutelin concentrations [ng/mL] quantitated by five
sandwich ELISA test kits using the R5, G12 or 401.21 (Skerritt) monoclonal antibodies
or two different polyclonal antibodies (pAb1 or pAb2)
Conclusions
Different gluten fractions yielded variable results within one ELISA test kit just like
different ELISA test kits yielded variable results within one gluten fraction. The mAbs
and pAbs showed different sensitivities and specificities towards wheat, rye and barley
and towards prolamin and glutelin fractions. Therefore, the gluten content was either
over- (up to 6-fold) or, more seriously for CD patients, underestimated (up to 8-fold).
A careful consideration of this variability of results between different ELISA test kits
is important, especially when analysing samples where the gluten source is unknown.
Aknowledgment
This research was funded by a grant of the German Coeliac Society (Deutsche
Zöliakie-Gesellschaft e. V.) awarded to Katharina Scherf in 2014.
References
1. Scherf KA, Poms RE. Recent developments in analytical methods for tracing
gluten. J Cereal Sci 2016; 67: 112-122.
2. Van Eckert R, Berghofer E, Ciclitira PJ, et al. Towards a new gliadin reference
material – isolation and characterisation. J Cereal Sci 2006; 43: 331-341.
3. Osman AA, Uhlig HH, Valdés I, et al. A monoclonal antibody that recognizes a
potential coeliac-toxic repetitive pentapeptide epitope in gliadins. Eur J
Gastroenterol Hepatol 2001; 13: 1189-1193.
4. Moron B, Cebolla A, Manyani H, et al. Sensitive detection of cereal fractions that
are toxic to celiac disease patients by using monoclonal antibodies to a main
immunogenic wheat peptide. Am J Clin Nutr 2008; 87: 405-414.
5. Skerritt JH, Hill AS. Monoclonal antibody sandwich enzyme immunoassay for the
determination of gluten in foods. J Agric Food Chem 1990; 38: 1771-1776.
6. Tye-Din JA, Stewart JA, Dromey JA, et al. Comprehensive, quantitative mapping
of T cell epitopes in gluten in celiac disease. Sci Transl Med 2010; 2: 41ra51.
7. Wieser H, Antes S, Seilmeier W. Quantitative determination of gluten protein
types in wheat flour by reversed-phase high-performance liquid chromatography.
Cereal Chem 1998; 75: 644-650.
8. Rallabhandi P, Sharma GM, Pereira M, et al. Immunological characterization of
the gluten fractions and their hydrolysates from wheat, rye and barley. J Agric
Food Chem 2015; 63: 1825-1832.
4.2 Progress towards the practical application of MS for
gluten detection and quantification
Michelle L. Colgrave1, Keren Byrne1, Malcolm Blundell2, Gregory J. Tanner2, Crispin
A. Howitt 2
1 CSIRO Agriculture Flagship, St Lucia, QLD 4067, Australia
2 CSIRO Agriculture Flagship, Black Mountain, ACT 2601, Australia
Introduction
Gluten is the collective name for a class of proteins found in wheat, rye, and barley.
Coeliac disease (CD) is an immune-mediated inflammatory disease of the small
intestine in a subset of genetically susceptible individuals that is triggered by the
ingestion of gluten, resulting in intestinal inflammation and damage. The only current
treatment for CD- and gluten-intolerants (70 million people globally) is lifelong
avoidance of dietary gluten. Gluten-free (GF) foods are now commonplace, however,
it is difficult to accurately determine the gluten content of GF products using current
methodologies as the antibodies are non-specific and show cross-reactivity. In
processed products measurement is further confounded by protein modifications
and/or hydrolysis. The development of mass spectrometry- (MS) based methodology
for absolute quantification of gluten is required for the accurate assessment of gluten,
including hydrolysed forms, in food and beverages to support the industry, legislation
and to protect consumers suffering from CD.
In this study, the hydrolysis of gluten was examined by proteomic profiling of beers
subjected to size-fractionation. Selected proteolytic and hydrolysed peptide fragments
were quantified by targeted mass spectrometry and the results were compared with
ELISA data. Strikingly, those beers containing high levels of hydrolysed B-hordeins
yielded potential false negatives by ELISA. The effect of the hydrolysed gluten
fragments on the suppression of ELISA response was further investigated revealing
interference that precludes the accurate quantification of beers using sandwich ELISA
technology. Secondly, proteomic profiling of gluten-enriched fractions of 16 cereal
grains including wheat, rye, barley, and oats focussing only on the grain (the edible
seeds) provided the foundation for the selection of peptide markers unique to wheat. A
rapid, robust, selective and sensitive method for detection of wheat contamination in a
range of cereals using multiple reaction monitoring (MRM) MS was developed and
applied to detect contamination in commercially available flour as well as in an
intentionally contaminated soy flour.
Materials and methods
Suppression of ELISA by hydrolysed gluten
A selection of beers were collected (60 commercial beers and a lab-brewed beer made
using barley cv. Sloop as listed in [1]) based on the stated ingredients or gluten
content. Each beer was either: left whole or applied to a 30 k or 10 k molecular weight
cut-off filter as described in [2]. The beers (whole and sub-30 k) were reduced by
addition of 10 μL of 50 mmol/L dithiothreitol (DTT) under N2 for 30 min at 60C. To
these solutions, 10 μL of 100 mmol/L iodoacetamide (IAM) was added and the
samples were incubated for 30 min at room temperature. To each solution, with the
exception of the 10 k fractions, 5 μL of 2 mg/mL trypsin was added and the samples
incubated at 37 C overnight. The digested peptide solution was acidified by addition
of 50 μL of 1% formic acid and stored at 4 C until analysis. All beer samples
generated were analysed by liquid chromatography (LC-) MS/MS and proteins
identified by automated database searching as described in [2]. Selected proteins (and
their peptide fragments) were further analysed by targeted proteomics employing
MRM MS [2].
Three beers were selected from the collection. Beers 6 and 31 gave a high and medium
response by ELISA, respectively. Beer 13 gave near-zero ELISA response, but
contained average levels of hordein by MS and high levels of hydrolysed gluten by
MS. Beer 13, either undiluted or diluted 2-, 5- or 10-fold was added to sandwich
ELISA wells containing beers 6 or 31 which had been diluted 1/50,000 or 1/5,000
respectively (so as to be in the middle of the Sloop hordein standard curve as described
in [3]). In a second experiment, aliquots of beer 13, either unfiltered or filtered through
100, 30, 10 or 3 k molecular weight cut-off centrifugal filters were added to sandwich
ELISA wells followed by aliquots of beers 6 or 31. All ELISA assays were processed
according to manufacturer’s instructions (ELISA Systems) and as described in [2].
Detection of wheat (gluten) contamination
Grains of wheat cv. Chara and 15 commercially relevant cereal grains were sourced as
described in [4]. A gluten-enriched fraction was prepared by dissolving wholemeal
flour (20 mg) in 200 μL 55% (v+v) propan-2-ol (IPA), 2% (w/v) dithiothreitol (DTT)
with incubation at 60 C for 30 min. The total protein extraction was performed by
dissolving wholemeal flour (20 mg) in 200 μL of 8 M urea, 2% (w/v) DTT with
incubation at RT for 30 min. The solutions were centrifuged for 15 min at 20,800 x g
and the supernatant was kept. The proteins were reduced, alkylated and digested using
trypsin [4] prior to LC-MS/MS analysis.
Results and discussion
Suppression of ELISA by hydrolysed gluten
We have previously demonstrated that barley-based beers that contain seemingly
average levels of hordein by MS have very low or zero readings by ELISA [5]. We have also demonstrated the existence of hordein peptide fragments in beers that have
been filtered through 10 k molecular weight cut-off filters with no enzymatic digestion
[1]. In this study, we sought to examine the relationship between the levels of
hydrolysed hordein in beer with the effect on the ELISA response.
LC-MS/MS analysis of barley-derived beers revealed that certain classes of hordein
were prone to hydrolysis (as judged by the percentage of each hordein present in the
30 k fraction relative to whole beer). Specifically, 57 + 12% and 37 + 7% hydrolysed
hordein were detected for B1- and B3-hordein, 31 + 13% for D-hordein compared to
18 + 10% for γ3-hordein. The resulting peptide fragments shared significant homology
with the immunotoxic epitopes determined for CD (Tab. 1). Strikingly, those beers
that contained high levels of B-hordein fragments gave near zero values by ELISA.
Table 1. Potential immunoreactive peptide sequences. Peptide sequences identified
(>95% confidence) in the sub-10 k fraction of 60 commercial beers. The sequences
are aligned with the closest matching immunoreactive epitope(s). Glutamic acid
residues shown in bold typefont (E) are produced by deamidation of glutamine (Q) by
the enzyme tissue transglutaminase, but are present as Q in the native protein.
Gluten fragments detected in the sub-10 k fraction contained epitopes that would likely
be recognized by the antibodies used in currently accepted ELISA assays. The Skerritt
antibody recognizes PQPQPFPQE & PQQPPFPEE, while the Mendez R5 antibody
recognizes QQPFP, QQQFP, LQPFP & QLPFP. Typical examples of gluten hydrolysis fragments detected include: B1-hordein (Uniprot: P06470 and I6SJ22)
peptide fragments QPQPYPQQPFPPQ and PQQPFPQQPPFG; B3-hordein (Uniprot:
I6SW30) peptide fragment QPQPYPQQPQPFPQ. QPQPYPQQPFPPQ shares 6/9 in
the DQ8 T cell epitope (EQPQQPFPQ) and PQQPFPQQPPFG shares 8/9 residues in
the DQ2 T cell epitope (QQPFPEQPQ) rendering them likely to possess
immunoreactivity.
The hydrolysed fragments that persist in beer show a dose-dependent suppression of
ELISA measurement of gluten despite using a hordein standard for calibration of the
assay. One drawback of sandwich ELISAs is they cannot adequately quantify gluten
that has been highly hydrolysed [6]. Sandwich ELISAs require two epitopes or
antibody binding sites. When a protein is hydrolysed, the various fragments may not
contain two epitopes. Many of the hydrolysed gluten fragments present in beer 13 have
one potential antigenic site. It was hypothesised that their binding to the capture
antibody precludes the binding of intact gluten proteins resulting in suppression of the
ELISA response. Beer 13 showed a concentration-dependent suppression of the
ELISA response (Fig. 1A, B) in both a high-gluten containing wheat beer (beer 6) and
a medium gluten-containing beer (beer 31). The suppression was demonstrated to be
due primarily to the <3 k fraction, i.e. hydrolysed peptide fragments (Fig. 1C, D).
Suppression was also caused by the high MW fraction (30 - 100 k) possibly the result
of aggregation (Fig. 1C, D).
Figure 1. Suppression of ELISA response by hydrolysed gluten present in whole beer.
Beer 13 (that contained high levels of hydrolysed gluten) was spiked into samples of
beers with differing levels of total gluten: (A) beer 6 (high); or (B) beer 31 (medium).
The columns represent (from left to right) no spike, spike with diluted beers (at either
10-, 5- or 2-fold dilutions) or the 2-fold diluted spike only. The mean A450 ± SD are
shown. Beer 13 was subsequently subjected to size-fractionation prior to addition in to
the same two beers: (C) beer 6; and (D) beer 31. Analysis of variance (ANOVA) was
carried out (2D: A and B; 1D, C and D). Within a group, columns with different letters
were significantly different by Tukey’s multiple comparison test (p ≤ 0.01)
Detection of wheat (gluten) contamination
In this study, 16 cereal grains that may be subject to contamination have been
comprehensively characterised using discovery proteomics to identify wheat-specific
peptide markers. As the focus of our work is the detection of gluten proteins, an
IPA/DTT extract that has been shown to preferentially solubilise the gluten fraction
[3,7] was used. In order to accurately identify the specific gluten isoforms present in
the extract, a combination of three proteolytic enzymes: trypsin, chymotrypsin and
thermolysin; was used to improve the protein sequence coverage (Tab. 2).
Table 2. Identification of HMW-glutenin isoforms was achieved using a combination
of three proteolytic enzymes by obtaining maximum sequence coverage.
The tryptic peptide products identified in the global LC-MS/MS analyses were
assessed to identify prototypic peptides that were unique to wheat and that were high
responding in the MS analysis, that is, peptides that yielded good peak area/intensity.
The peak area/intensity is dependent on the ionisation efficiency of the peptide, but
also the abundance of the protein and, therefore, the peptide and the efficiency of
proteolytic digestion under the experimental conditions. The top 20 highest responding
peptides were selected and subjected to BLASTp analysis (NCBI BLASTp server)
against all other cereals to ensure specificity to wheat based on protein sequences that
exist in the public databases. As not all of the grains examined have been sequenced at
the genome level, it was expected that the public databases would be incomplete. To
ensure the selected peptide markers were wheat-specific, the 16 grain extracts were
screened using the LC-MRM-MS method developed herein. Peptide MRM transitions
that showed interference, broad peaks and/or low intensity peaks were excluded from
further analysis. The 10 best wheat peptides were selected for subsequent MRM
analyses.
Figure 2. From the top 10 wheat-specific peptide markers (A), the best 4 were selected
(B) and allowed the detection of wheat contamination in rye (C) and millet (D) flour.
Detection of contamination in commercial products: (E) Flour from rye, millet,
sorghum, buckwheat, and oats were confirmed with low level wheat contamination
Fig. 2 shows the LC-MRM-MS analysis targeting the wheat-specific peptide markers.
The total ion chromatogram (TIC, Fig. 2A) shows ten peptide markers (labelled P1-
P10) from which peptides P3, P4, P7 and P9 were selected and are shown in the
extracted ion chromatogram (XIC, Fig. 2B). Fig. 2E shows the results of the LCMRM-
MS analysis of 16 grains using four wheat-specific peptide markers. As
expected, the markers were present in high abundance in wheat, green wheat (Freekeh)
and spelt. However, all four peptide markers were also detected in rye (average MRM
peak area 4.2% relative to wheat), in millet (0.3%), and buckwheat (0.02%), whilst three of the four peptide markers were detected in sorghum (0.03%) and oats (0.05%).
The XIC for the analysis of pre-milled rye (Fig. 2C) and millet (Fig. 2D) flour
revealed peaks matching to all four peptide markers (P3 at 3.6 min; P4 at 4.0 min; P7
at 5.5 min; and P9 at 7.3 min) indicating that the commercial flour samples of both rye
and millet were contaminated by wheat (4.2% in rye and 0.3% in millet).
Uncontaminated whole grain of rye and millet were obtained, visually inspected,
milled to fine flour after careful cleaning of the mill, analysed and shown to be devoid
of wheat confirming the detection of the peptide markers was due to wheat
contamination and not the presence of endogenous proteins. Trace levels of the wheat
peptide markers were also observed in commercial flours from oats, sorghum, and
buckwheat, but the levels detected were <0.05% relative to wheat.
Figure 3. Peptide markers are useful for diverse wheat varieties. The wheat peptide
markers were assessed across 14 commercial wheat lines (A) and nine MAGIC parent
lines (B)
The wheat peptide markers were selected from analysis of a single wheat cultivar
Chara. In order to assess the utility of the wheat peptide markers in broader
applications, we investigated their presence and level in 14 commercially available
wheat cultivars and in nine parent lines of the 4 and 8 way MAGIC populations
developed by CSIRO [8], that are thought to represent 80% of the genetic diversity
within the bread wheat genome (Fig. 3). Variation in the peptide levels of 22-26% for
the 14 commercial lines and 11-16% for the nine MAGIC parent lines for P4, P7 and
P9 was observed. Only one peptide (P3) was absent in the wheat cultivar Xioayn, a
Chinese winter cultivar used for noodles, and showed higher variation between
cultivars (43-57%). Overall, these data demonstrate that the selected peptide markers
are suitable for detection of wheat across a wide variety of wheat representative of
those used commercially.
Conclusions
Analysis of barley-derived beers revealed that high levels of gluten (B-hordein)
hydrolysis correlated with low values by ELISA. The hydrolysed fragments that
persist in beer caused a dose-dependent suppression of ELISA gluten measurement.
Global proteomic analysis of 16 economically important cereals utilising SDS-PAGE,
Western blotting and LC-MS/MS was used to characterise the “gluteome” and select peptide markers specific to gluten and/or wheat for targeted quantitative MS assays.
Wheat-specific peptide markers were detected in 14 wheat varieties that together
constitute 80% of modern wheat genetic diversity and facilitated the detection of
wheat contamination in commercial flours.
References
1. Colgrave, ML, Goswami H, Howitt CA, et al. What’s in a beer? Proteomic
characterisation of hordein (gluten) in beer. J Proteome Res 2012; 11: 386-396.
2. Colgrave, ML, Goswami H, Blundell M, et al. Using mass spectrometry to detect
hydrolysed gluten in beer that is responsible for false negatives by ELISA. J
Chrom A 2014; 1370: 105-114.
3. Tanner GJ, Blundell MJ, Colgrave ML, et al. Quantification of hordeins by
ELISA: The correct standard makes a magnitude of difference. Plos ONE 2013; 8:
e56456.
4. Colgrave, ML, Goswami H, Byrne K, et al. Proteomic profiling of 16 cereal
grains and the application of targeted proteomics to detect wheat contamination. J
Proteome Res 2015; 14: 2659-2668.
5. Tanner GJ, Colgrave ML, Blundell MJ, et al. Measuring hordein (gluten) in beer– A comparison of ELISA and mass spectrometry. Plos ONE 2013; 8: e56452.
6. Thompson T, Mendez E. Commercial assays to assess gluten content of glutenfree
foods: Why they are not created equal. J Am Diet Assoc 2008; 108: 1682-
1687.
7. Ribeiro M, Nunes-Miranda JD, Branlard G, et al. One hundred years of grain
omics: identifying the glutens that feed the world. J Proteome Res 2013; 12: 4702-
4716.
8. Huang BE, George AW, Forrest KL, et al. A multiparent advanced generation
inter-cross population for genetic analysis in wheat. Plant Biotechnol J 2012; 10:
826-839.
4.3 Studies on the analysis of gluten-containing cereals
Kathrin Schalk, Katharina Scherf, Peter Koehler
Deutsche Forschungsanstalt für Lebensmittelchemie, Leibniz Institut, Freising,
Germany
Introduction
Coeliac disease (CD) is an inflammatory disorder of the upper small intestine caused
by the ingestion of gluten proteins from wheat (gliadins, glutenins), rye (secalins),
barley (hordeins), and possibly oats (avenins). The only effective therapy for CD
patients is a strict gluten-free diet by consuming gluten-free foods, which contain less
than 20 mg gluten/kg [1]. To ensure the safety of gluten-free products for CD patients,
it is essential that appropriate analytical methods with high specificity and sensitivity
are available. The most commonly used method for gluten analysis is an enzymelinked
immunosorbent assay (ELISA). Currently, the ELISA based on the R5
monoclonal antibody is endorsed for gluten analysis in maize-matrices [2] and is
defined as type I method [3]. In general, with ELISA it is only possible to determine
the prolamin content in foods, and not the whole gluten content. The gluten content is
then calculated by multiplying the prolamin content by a factor of 2. This calculation
is based on the assumption that the ratio of prolamins to glutelins is 1. The problem is
that different types of grains contain different proportions of prolamins and glutelins.
As a result, the gluten content may be either over- or underestimated. In particular the
latter is problematic for CD patients [4].
Therefore, a new independent non-immunochemical method for the quantitation of
prolamins and glutelins (= total gluten) is urgently needed to verify the results
determined by ELISA. Liquid chromatography-tandem mass spectrometry (LCMS/
MS) appears to be a suitable method for the quantitation of gluten.
Therefore, the aim of this study was to identify marker peptides from wheat, barley,
rye, and oats to develop a stable isotope dilution assay for the quantitation of intact and
partially hydrolysed gluten.
Materials and Methods
Defatted flours from wheat, barley and oats (a mixture of four cultivars of each grain,
harvest 2013) were extracted by a modified Osborne procedure [5]. First,
albumins/globulins were extracted with 0.067 mol/L K2HPO4/KH2PO4-buffer with 0.4
mol/L NaCl (pH = 7.6) at 20 °C. Second, the residue was extracted with 60% (v+v)
ethanol at 20 °C to obtain the prolamin fraction. Finally, the glutelin fraction was
extracted with 50% (v+v) 1-propanol/urea/Tris-HCl/dithiothreitol (pH = 7.5) at 60 °C.
Albumins/globulins were discarded because they were not relevant for gluten analysis. Different protein types were isolated using preparative reversed-phase highperformance
liquid chromatography (RP-HPLC). Gliadins (wheat) were separated into ω5-, ω1,2-, α-, and γ-gliadins, and glutenins (wheat) were separated into lowmolecular-
weight- (LMW-GS) and high-molecular-weight glutenin subunits (HMWGS).
Barley prolamins were divided into γ- and C-hordeins, and barley glutelins into
B- and D-hordeins. Furthermore, all isolated protein types and fractions were
characterized by sodium dodecylsulphate-polyacrylamide gel electrophoresis (SDSPAGE)
to verify the molecular weights and the purity of the preparations.
In order to be able to develop a LC-MS/MS method for gluten proteins, partially
hydrolysed reference proteins were necessary. For this purpose, the isolated reference
proteins (protein types, protein fractions) as well as the flours were incubated with
chymotrypsin for 24 h at 37 °C and pH 7.8 (ratio enzyme:protein; 1:200). After
incubation, the peptide mixtures were purified by solid phase extraction on DSC-18
cartridges. Then, the hydrolysates were analysed by LC-MS/MS.
Results and Discussion
Prolamin and glutelin fractions from wheat (gliadins/glutenins) and barley (hordeins)
were separated into protein types by preparative RP-HPLC. Avenins were not further
separated. As an example, Fig. 1 shows the chromatogram of the glutenins, which
were separated into HMW- and LMW-GS. All isolated protein types were characterrized
by RP-HPLC and SDS-PAGE to verify the range of the molecular weights.
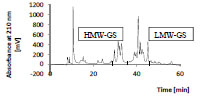
Figure 1. Preparative RP-HPLC of isolated wheat glutelins (glutenins; 25 mg/mL,
injection volume: 300 μL). HMW-GS, high-molecular-weight glutenin subunits; LMWGS,
low-molecular-weight glutenin subunits
All isolated protein types from wheat, barley, and oats were hydrolysed and analysed
by LC-MS/MS to identify gluten marker peptides which will be used for gluten
quantitation. These marker peptides must fulfil specific requirements to be suitable for
gluten quantitation. Firstly, the amino acid sequences must be characteristic for each
protein type. Ideally, the peptide sequences should not occur in other protein types of
gluten or other proteins. Secondly, the marker peptides should have a peptide length of
8 to 20 amino acids, because too small peptides are not specific enough and peptides
longer than 20 amino acids are unsuitable for quantitation due to high complexity and the large number of fragments. Thirdly, the marker peptides should not contain
cysteine residues because of their tendency to oxidation.
To define gluten marker peptides, all isolated protein types from wheat and barley
were hydrolysed and analysed by LC-MS/MS in the first step. By using the NCBIDatabase
(National Center for Biotechnology Information) and the MASCOT-software
(Matrix Science, London, UK), gluten peptides were identified. In the second step, the
criteria for gluten marker peptides were applied to get candidate peptides. To verify
this selection, all protein fractions from wheat, barley, and oats as well as the flours
were hydrolysed and analysed by LC-MS/MS. Finally, peptides which were identified
in all hydrolysates (protein type, protein fraction and flour) and which fulfilled the
requirements were suitable for gluten quantitation. For each protein type, two or three
gluten marker peptides were defined (Tab. 1 and Tab. 2). For ω5-gliadin, only one
marker peptide was defined because of the low amount in flour.
Table 1. Selection of marker peptides for wheat gluten, their mass-to-charge-ratios,
and the peptide scores, which were detected in flour.
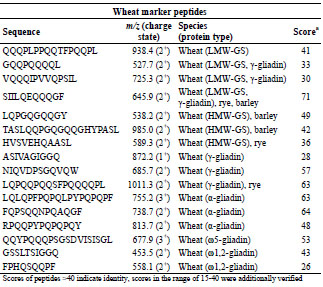
In general, it was also an important criterion to select marker peptides with a high
score to increase the chance to detect them in different food matrices. Although the oat
peptide DPSEQYQPYPEQQEPF was detected with a low protein score of 15, it was
defined as marker peptide because it contains a deamidated T cell epitope
(PYPEQEQPF) (Tab. 2) [6].
Table 2. Selection of marker peptides for gluten from barley and oats, their mass-tocharge-ratios, and the peptide scores, which were detected in flour.
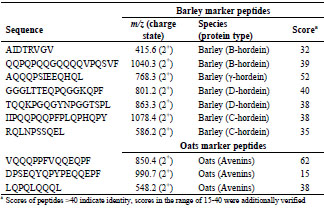
Conclusions
The LC-MS/MS analysis of hydrolysed proteins from wheat, barley and oats yielded a
number of peptides which can be used as gluten marker peptides. Peptides, which were
identified in hydrolysed protein types, protein fractions as well as the flour, were
selected as marker peptides. For each protein type, two to three marker peptides were
defined, which provide the basis to develop a non-immunochemical independent
method for gluten quantitation.
References
1. Wieser H, Koehler P. The biochemical basis of celiac disease. Cereal Chem 2008;
85(1): 1-13.
2. Koehler P, Schwalb T, Immer U, et al. AACCI approved methods technical
committee report: collaborative study on the immunochemical determination of
intact gluten using an R5 sandwich ELISA. Cereal Foods World 2013; 58: 36-40.
3. Codex Standard 324-1999, 2014. Recommended methods of analysis and
sampling. Codex Alimentarius Commission. Amendment 4.
4. Wieser H, Koehler P. Is the calculation of the gluten content by multiplying the
prolamin content by a factor of 2 valid? Eur Food Res Technol 2009; 229: 9-13.
5. Wieser H, Antes S, Seilmeier W. Quantitative determination of gluten protein
types by reversed-phase high performance liquid chromatography. Cereal Chem
1998; 75 (5): 644-650.
6. Londono DM, van’t Westende WPC, Goryunova S, et al. Avenin diversity
analysis of the genus Avena (oat). Relevance for people with celiac disease. J
Cereal Sci 2013; 58: 170-177.
4.4 Strategies to reduce or prevent wheat coeliacimmunogenicity
and wheat sensitivity through food
Luud J.W.J. Gilissen1, Ingrid M. van der Meer1, Marinus J.M. (René) Smulders2
1 Wageningen UR, Bioscience, Wageningen, The Netherlands
2 Wageningen UR, Plant Breeding, Wageningen, The Netherlands
Abstract
Cereals are among the oldest foods of humans. Wheat is one of these. In present times,
several syndromes are, whether true or false, increasingly attributed to the consumption
of wheat, with increasing costs for medical care and decreasing turnover for the
food industry, especially the bakery sector. Many western societies show remarkable
annual increases in their health care costs, often surmounting their economic growth
rates. Governmental health policies should urgently revert towards the stimulation of
disease prevention practices instead of maintaining the stimulation of expensive
medical care.
Here we review and discuss possible strategies to prevent or reduce the incidence of
wheat-related conditions through application of breeding and food-related
technologies. Breeding includes selection and crossing for low-immunogenic wheat
varieties using varieties, accessions, and wild relatives, silencing the expression of
gluten genes, and advanced genome editing techniques to eliminate gluten genes, such
as CRISPR/Cas9 technology. Food-related approaches include the reduced application
of vital gluten, exclusion of gliadin from isolated gluten by separation, increased use
of sourdough fermentation and malting, utilisation of patient-specific gluten epitopesensitivity
profiles, introduction of the gluten contamination elimination diet (GCED)
especially in individuals that are non-responsive to the gluten-free diet, to acquire
more fundamental knowledge on immune modulating factors, and the design of an
intervention study to learn about the medical and mental motives of people to switch
towards a ‘gluten-free’ diet. Finally, we discuss the development, testing and
promoting of efficient disease prevention measures within the societal context.
Introduction
Agriculture started some 10,000 years ago. It is a myth, however, that the consumption
of cereal grains by humans begun only at that time. The divergence of early
humanoids from man-apes occurred about 6 My ago with leaving the African forest
and moving into the savannah areas. Concomitantly, the humanoids increased their
feeding on small and hard grass (cereal) seeds. This has been concluded from the
shape of the molars and the increased thickness and the molecular composition of the
enamel [1-3]. From 1.5 My ago onwards, Homo species increased the fraction of C4-
based plant resources (i.e. cereals) in their diet as was also concluded from enamel analysis [4]. As early as 120,000 years ago, during an interglacial period, the Levant,
where barley and wheat are endemic species, was a fruitful settling and meeting place
where West-European Neanderthals exchanged genes with early modern humans [5].
Neanderthals from the Iraq region are known to have consumed cooked Triticeae
grains, especially barley, about 50,000 years ago [6]. Excavations from 32,600 years
ago in Italy revealed the occurrence of thermal pre-treatment and grinding of cereal
seeds (oats) by humans [7] indicating that cereal food technology must have been
developed much earlier. From more recent times, some 15,000 years ago, remainders
from barley groat meals and porridge have been found, as well as remains from
unleavened bread from barley flour [8]. Further, data from 11,000 years ago show
ground collection of wild barley and wild emmer wheat seeds as an intermediate step
between seed collection by hunter-gatherers and cereal harvesting by early farmers [9].
Agriculture and cereal (barley and wheat) consumption, therefore, did not appear
suddenly 10,000 years ago, but had a long and gradual origin.
Remarkable in this apparently long tradition of grain consumption by humans is the
development of bread wheat and spelt wheat (both with the AABBDD genome). These
wheats are considered as the natural hybrids between (early cultivated) emmer wheat
(with AABB genome) and wild Triticum tauschii (with the DD genome). The
hybridization is suggested to have occurred some 8,000-9,000 years ago, maybe as a
side-result of early agricultural activity [10]. More recently, 2,000 years ago, coeliac
disease (CD) was identified (but not yet directly related to the consumption of wheat,
barley and rye) and received its name from Arathaeus of Cappadocia [11].
Undoubtedly, this disease must have existed much longer and most probably at
significant frequency.
In the 20th century cereal breeding and cereal food processing have become highly
advanced. Wheat and barley can be grown now also at high latitudes and large
acreages. Millers are able to refine cereal meal and separate many fractions that can be
used in innumerable applications. Simultaneously, the prevalence of and mortality due
to undiagnosed CD increased four times [12]. During the last years, non-celiac
wheat/gluten sensitivity (NCWGS) seems a new condition, although some case reports
were already known from the seventies of last century [13,14]. A recently estimated
prevalence in the general population of NCWGS, obtained from indirect evidence, is
slightly more than 1% (which is similar to the prevalence of CD in the general
population [15]). NCWGS is mostly reported to occur in females in the age group of
30-50, although also paediatric cases are known. In spite of the generally low
estimated prevalence of both CD and NCGWS, more than 10% of the adults in the
USA and the UK changed their diet towards gluten-free, mainly on the basis of selfdiagnosis
[15]. The situation may be even more extreme in the USA, where 30% of
restaurant visitors demand gluten-free food [16]. Nevertheless, in NCWGS a direct
relationship with gluten itself or with other wheat-related compounds like amylase
trypsin inhibitors (ATI), lipid transfer proteins (LTP), fermentable sugar compounds
(FODMAPS), or other compounds or factors as causing agents remains unclear [15].
This situation is different for CD. While the relationship with gluten consumption has been established clearly and is well-understood, 80-90% of the CD patients has not yet
been diagnosed or has been diagnosed wrongly. This large part of the CD population
maintains the daily consumption of gluten-containing foods, unaware of the risk of
worsening their health status. These figures together show an underestimated
population of people with CD, and a self-overestimated population of NCWGS people,
both following a (strict) gluten-free diet.
In this short review we propose a broad array of strategies to meet the desire of CD
and NCWGS populations and individuals for safe(r) cereal food (see also [17-19]).
These strategies fall into two categories: ‘plant-related strategies’ that use various
advanced breeding technologies for the reduction of the occurrence of CD epitopes in
especially gliadins, and ‘food-related strategies’ that focus on targeted processing to
enable gluten avoidance.
Plant-related strategies
Selection
Selection of low CD-toxic wheat varieties and accessions has been performed using
epitope-specific monoclonal antibodies (mAbs): out of hundreds of such lines only a
few gave a relatively low mAb response [20,21]. The utility of screening with epitopespecific
mAbs is limited as they do not recognize the complete epitope (mAbs
recognize a maximum linear sequence of six amino acid residues, whereas the T cell
epitope is nine amino acids long) and the signal may be ambiguous and not
quantitative (i.e., also responding to non-intact epitope sequences). A direct approach
combining deep sequencing of the N-terminal region of gliadin transcripts as a prescreening
of developing seeds of a single variety, followed by quantitative proteomics
of ripe seeds [22] enabling simultaneous quantification of several CD epitopes is much
more straightforward and conclusive.
Traditional breeding
In barley, a reduction of the gluten (hordein) content to below 5 mg/kg (the threshold
for gluten content in ‘gluten-free’ products in the Codex Alimentarius is 20 mg/kg)
was achieved using traditional breeding strategies by combining three recessive
alleles, which act independently of each other to lower the hordein content in the
parental varieties. Further breeding was applied to increase the grain size to near wildtype
levels. The plants were agronomically acceptable and the produced grains showed
good malting characteristics and brewed successfully. The advantage in traditional
breeding of barley over bread wheat is its diploid genome level and the occurrence of
only four hordein protein families [23].
Synthetic hexaploids
The D-genome of bread wheat contributes most to CD-immunogenicity with the
highest number and diversity of epitopes [24], but shows the lowest degree of variation
compared with the A- and the B-genome. Newly created synthetic hexaploids carrying a diversity of D-genomes are now in a screening program which aims to identify
hexaploid wheat lines with reduced CD-immunogenicity [18].
Deletion of loci
A ‘deletion line’ lacking a large part of the short arm of chromosome 6D, which
eliminated the 6D alpha-gliadin locus, showed strongly decreased mAb responses
against the Glia-alpha1 and Glia-alpha3 epitopes. Changes in dough mixing properties
of this line could be compensated by the addition of oat prolamins (avenins), which are
not CD-immunogenic. Such deletion lines are especially useful as model systems but
lack economic value as they grow poorly. This is probably an effect of the loss of
hundreds of other genes on this chromosome arm [25].
RNAi
Two different approaches have been applied to reduce the amount of gliadins in wheat
through silencing of gliadin gene expression using RNA interference (RNAi). In the
first approach, the gliadin genes themselves were targeted directly and successfully
[26-31]. Flour made from grains in which gliadins were silenced showed no T cell
elicitation, but retained baking quality [30]. The other approach used RNAi to suppress
homologs of the DEMETER (DME) gene. This gene encodes a 5-methylcytosine
DNA glycosidase which demethylates the promoter region of gliadin and LMW
glutenin genes in wheat endosperm, a step that is necessary to switch on the expression
of these genes during endosperm development. Suppression of expression of DME
resulted in >75% reduction in the amount of immunogenic prolamins [32].
Mutation breeding and genome editing
Mutation breeding is a relatively old technology using chemical (especially ethyl
methane sulfonate, EMS) or ionizing irradiation (e.g. gamma-radiation, fast neutrons)
to introduce random mutations in the DNA. Mutation selection on populations of
mutant progenies can be carried out through Targeting Induced Local Lesions in
Genomes (TILLING), a technology based on molecular DNA sequence analysis
followed by bioinformatics analysis. It is expected that no single line from such an
experiment would exist in which all gliadins have been mutated simultaneously, but
this strategy may be used in combination with other strategies mentioned above.
Precise genome editing recently sees a stormily increasing interest as the
CRISPR/Cas9 technology enables inducing mutations and deletions at specific,
targeted locations within the genomes of animals as well as plants. Several groups
currently apply CRISPR/Cas9 in wheat, but no results have been published yet.
Food-related strategies
Reduction of vital gluten
Vital wheat gluten is a major side-product in the production of wheat starch which is
the raw material for further production of native and modified starch, glucose syrup,
liquid and crystalline polyols, maltodextrins, all with high-impact applications in the
food industry. Wheat starch is also used for the production of alcohol, including
bioethanol. Since wheat starch is economically the most important product from wheat
(much more important than flour for bakery purposes), the production of its sideproduct,
vital gluten, is enormous. Vital gluten is increasingly applied as protein
additive in food products for technological improvement. The large-scale addition of
vital gluten to a wide range of processed food products has contributed to the increase
in total gluten consumption: between 1977 and 2007, gluten intake in the western
world has tripled [33], and most of this increase took place after 2000. The increase
relates only partly to bread and bakery products, where vital gluten is applied to
maintain high loaf volumes, but is mainly the result of its use in the form of ‘hidden’ ingredient, often not labelled, in a large number of food products. Currently, a
movement is going on in the modern bakery in The Netherlands towards ‘back to
basics’. This includes ‘clean label’ strategies and avoidance or strong reduction of the
application of bread quality-improving ingredients such as enzymes and vital gluten in
artisanal and industrial whole grain breads. There are, however, no indications of
reduced application of vital gluten in other sectors of food production. On the contrary,
more and more vital gluten application in foods is occurring. In addition, industrial
conversion of wheat starch into other food-grade carbohydrates will not eliminate all
stable and potentially immunogenic gluten. Therefore, foods containing these modified
carbohydrates may still contain low levels of gluten. Together these processes have led
to a steady increase of gluten accumulation in the general daily diet.
Elimination of gliadins from gluten
HMW-glutenins give dough its elasticity, while the gliadins can be replaced by other
proteins, e.g. by the coeliac-safe avenins, for maintaining good baking quality [25].
The HMW-glutenins also have a relatively low coeliac-immunogenicity. This means
that separation of glutenins from total gluten is a realistic option that may contribute to
new applications in foods aiming at a strongly reduced immunogenic gluten load.
Nearly complete separation can be achieved at lab scale [34]. Industrial upscaling to
economically and technologically viable levels seems to be more recalcitrant [35], and
would need further research.
Sourdough fermentation
In Germany, the prevalence of CD (measured using a single antibody test) was found
to be remarkably low: 0.4% and 0.2% in women and men, respectively [36], which
confirmed earlier data showing 0.1-0.4% prevalence on the basis of anti-tTG and EMA
positivity [37]. Recent estimations of the CD prevalence in Germany go up to 0.8%, especially in children up to 17 years old [38]. The authors suggest that this may reflect
underdiagnosis in earlier studies, but another explanation is also possible.
Germany has a longstanding tradition of sourdough bread consumption. Sourdough
fermentation has been suggested to enable the manufacture of ‘gluten-free’ or ‘low-ingluten’ wheat-based products through proteolytic gluten degradation [39]. More
recently, a two-step hydrolysis including fungal peptidases and Lactobacillus endopeptidase
activity, respectively, resulted in a wheat flour-derived product with a gluten
content below 10 mg/kg. This product appeared not harmful to individuals with CD
[40]. These observations are promising, but need further research on the potential of
sourdough products in a safe ‘gluten-free’ diet for individual cases. For this, first a
strict definition of the type of sourdough is required and the process of gluten
degradation needs to be analysed in detail. Further, the question is whether large-scale
consumption by the general population of well-defined sourdough products can indeed
contribute to the reduction of the incidence and symptom severity of CD and NCWGS.
The answer requires large-scale epidemiologic research. If sourdough indeed reduces
the exposure to intact gluten epitopes, then the recent increase in prevalence of CD in
young people may in fact reflect a change in the diet of German children and
adolescents towards fast food consumption (white and yeast-based bread).
Malting
This process is not only relevant for brewing, but has also been applied since ages in
the bakery sector. Endogenous proteolytic enzymes become active during malting, i.e.
the first steps in seed germination, to produce amino acids from the storage proteins in
the endosperm as building blocks for new structural and house-keeping proteins for the
young growing seedling. During this germination process, which lasts several days
until the seed has been transformed into a seedling, gliadins may become degraded
first. This means that (specific) gliadinases will show early activity. Application of
enzyme supernatants from a small stock of germinated seeds (especially barley seeds)
have recently been suggested to be applicable in large-scale production of flour
reduced in prolamin content [41,42]. In addition, it has been shown that germinated
and fermented rye and wheat sourdough effectively degraded 99.5% and 95% of the
prolamins, respectively [43,44].
Patient-specific epitope-sensitivity profile
Genomic data of plant species and cultivars are rapidly increasing in number and
detail; this also includes the wheat genome (with the sequences of dozens of gluten
(gliadin) genes and epitopes and their expression profiles). Similarly, genomic data of
humans, also at the individual level, are laying the basis for personalized food and for
personal health and medical strategies. Individuals with CD appear to have different
and epitope-specific sensitivities to gluten [45,46]. Combining both these sets of
gliadin (gluten) and human genomics data may reveal possibilities to further
investigate wheat varieties with epitope profiles that may fit to the specific sensitivity
profile of an individual patient or specific groups of patients.
Gluten contamination elimination diet (GCED)
Some coeliac patients have persistent symptoms and villous atrophy despite strict
adherence to a gluten-free diet. They are referred to as having ‘non-responsive CD’, a
subset of which may have true ‘refractory CD’. Some non-responding CD patients
simply react to minor amounts of gluten, which may be present as contamination in
regular, processed gluten-free foods. As mentioned above (in the paragraph on ‘Reduction of vital gluten’) foods labelled as gluten-free may contain minor amounts
of gluten or stable gluten fragments due to their introduction into these food products
through complex food processing. This may even happen in increasing numbers. Most
(over 80%) of the non-responsive patients, however, improved on a so-called ‘gluten
contamination elimination diet’ (GCED) that included only fresh and unprocessed
foods. These patients appeared not to suffer from ‘refractory CD’ and were able to
return to a traditional gluten-free diet without return of symptoms [47].
Immune-modulating factors
The high frequency of individuals sensitised to a broad range of cereal (i.e. wheat)
proteins led to the suggestion that wheat (and related cereal) foods may have high
immunogenic potential, perhaps greater than other foods in general, although without
any occurrence of clinical symptoms [48]. We hypothesised that the consumption of
wheat positively influences the maturation of the immune system in early childhood in ‘cross-talking’ with the intestinal microbial community [49]. This may especially be
relevant in populations not yet affected by the ‘western lifestyle’ with its increased
hygiene [50], and not yet saddled with the related ‘western lifestyle syndrome’ characterised by disturbed immune and (intestinal) microbial functioning and
increased susceptibility to chronic inflammatory diseases. Western urban
environments often lack many of the microbes with which humans have co-evolved,
and which may function as inducers of immune-regulatory circuits [51,52]. Integration
of research on factors related to gut microbiota (including foetal programming by
maternal microbial exposure, neonatal programming and hygiene), on diet and lifestyle
factors, and on human genetics and epigenetics, will hopefully reveal some of the
immune-regulatory environmental factor(s) to explain the recent increase in immunerelated
diseases. Many studies have been conducted to identify the features of a ‘healthy microbiome/microbiota’ and the alterations on the host-microbiota cross-talk,
promoting the progress from health to disease in diverse disorders. These studies show
the enormous complexity and challenges of this scientific arena. Advances could open
ways to the development of microbiome-informed strategies for (personalised)
preventive and therapeutic measures including dietary strategies that help optimise the
partnership between the gut microbiota and host immunity, increasing microbiome
homeostasis in, and health resilience of, the host [53]. The manipulation of the gut
microflora is in its infancy but may have enormous potential for the future with regard
to prevention of immune system-mediated diseases [51,52]. How intestinal microbiota
may play a role in the development of the immune system was demonstrated during a
helminth therapy (through experimental hookworm infection), showing a significant increase in microbial species richness and, interestingly, a promoted tolerance in
coeliac subjects to escalating gluten challenges [54,55]. Helminth infections are known
to be correlated with a Th2 response [56]. In this regard, measurement of IgE or other
Th2 markers might be of interest to demonstrate a possible reversion of the immune
system away from autoimmunity (i.e. away from CD).
Health Grain Forum Intervention Study
The above refers to an increasing but still small percentage of the population. There
are currently solid grounds to advise the general consumer, i.e., the majority of the
population, to consume whole grain wheat products. Very large cohort studies clearly
demonstrate that consumption of whole grain products, including wheat and especially
the cereal bran fraction, significantly reduces the risk of several chronic diseases
[57,58]. These diseases are common, and therefore reducing these risks has a major
effect on life expectation and wellbeing. Nevertheless, worldwide, an anti-wheat and
anti-gluten hype has developed over the last five years, with significant impact on all
parts of the cereal supply chain and concomitantly significantly reduced sales of bread,
breakfast cereals and pasta products in various markets. The question arises why so
many people (≈30% in the USA, 15% in Australia, and increasing numbers in other
Western countries) seemingly feel more comfortable on a gluten-free or wheat-free
diet. Hard data about negative human health effects (beyond CD and wheat allergy)
are lacking, although popular books such as ‘Wheat Belly’ [59] and ‘Grain Brain’ [60]
suggest differently. In these books, the cereal supply chain is blamed for feeding the
world with sick-making food, but several of the arguments used are objectively wrong
[61,62]. The clinical picture of NCWGS is variable and usually includes IBS-like
gastrointestinal manifestations and neurological symptoms, such as foggy mind and
headache [15]. Expert criteria for the diagnosis of NCWGS have been recommended,
but specific and sensitive biomarkers for NCWGS are lacking [63]. Health Grain
Forum now proposes to perform a double-blind placebo-controlled human intervention
study addressing the effects of different wheat species, their components, and various
processing steps towards end products. This study aims at measuring effects on human
metabolism and health parameters to obtain reliable data useful for future human
dietary recommendations and appropriate food processing and product development.
In vivo effects, to be measured in this study, are related to gut feeling and adverse
effects, analysis of gut microbiota and colonic metabolism, and to gut permeability.
The intervention will also include the measurement of nocebo effects on the
consumer’s perception after consumption. The intervention will include a wellcontrolled
cohort of IBS patients. A consortium of research organisations and
stakeholder cereal food companies has recently been established. The project may start
in 2016 [64].
The societal context
Health care costs in The Netherlands (with 17 million inhabitants) amounted to almost
100 B€ in 2015, with an estimated annual increase of 6%, which is much higher than
the total Dutch economic growth. With this amount, the Dutch spend per capita almost
as much as a citizen of the USA, which is the highest in the world. The spectacular rise
of these costs is partly due to an increasing elderly population, to increasing prices of
special medical treatments in health care, and last but not least to the enormous
increases in the prices and in the applications of medicines. In this regard, the
pharmaceutical industry plays a dubious role [65]. Remarkably, in Cuba, the health
care budget per capita is 20 times lower compared to the USA, however, with an equal
life expectation. People have argued that part of the health care budget should be bent
towards the development, testing and promotion of efficient prevention measures. We,
therefore, argue that also ‘big food industries’ should take a responsibility for the
health status of the population.
Conclusion
The societal context demonstrating increasing costs for health care should strongly
urge national governments to reconsider their health care policies and change these
into disease prevention policies with a focus on the maintenance of the health of its
individual people. In this paper, we give a variety of examples for plant and foodtechnological
related strategies that may contribute as small steps towards the
reduction of the incidence of remarkable food-induced syndromes, specifically CD and
NCWGS, caused by the consumption of one of our oldest and most frequently
consumed foodstuffs worldwide.
Acknowledgements
The authors are grateful to the EU 7th Framework Programme project ‘Traditional
Food Network to improve the transfer of knowledge for innovation’ (TraFooN) (grant
agreement no: 613912) that partially supported writing of this paper.
References
1. Strait SG. Tooth use and the physical properties of food. Evol Anthropol 1997; 5:
199-211.
2. Sponheimer M, Lee-Thorpe JA. Isotopic evidence for the diet of an early hominid,
Australopithecus africanus. Science 1999; 283: 368-370.
3. Senut B, Pickford M, Gommery D, et al. First hominid from the Miocene
(Lukeino formation, Kenia). Compt Rend Acad Sci Series IIA 2001; 332: 137-144.
4. Cerling TE, Manthi FK, Mbua, et al. Stable isotope-based diet reconstructions of
Turkana Basin hominins. Proc Natl Acad Sci 2013; 110: 10501-10506.
5. Kuhlwilm M, Gronau I, Hubisz MJ, et al. Ancient gene flow from early modern
humans into Eastern Neanderthals. Nature 2016; doi:10.1038/nature16544.
6. Henry AG, Brooks AS, Piperno DR. Microfossils in calculus demonstrate
consumption of plants and cooked foods in Neanderthal diets (Shanidar III, Iraq;
Spy I and II, Belgium). Proc Natl Acad Sci 2011; 108: 486-491.
7. Lippi MM, Foggi B, Aranguren B, et al. Multistep food plant processing at Grotta
Paglicci (Southern Italy) around 32,600 cal B.P. Proc Natl Acad Sci 2015; 112:
12075-12080.
8. Eitam D, Kislev M, Karty A, et al. Experimental barley flour production in
12,500-year-old rock-cut mortars in Southwestern Asia. PLoS ONE 2015;
10:e0133306. Doi:10.1371/journal.pone.0133306.
9. Kislev ME, Weiss E, Hartmann A. Impetus for sowing and the beginning of
agriculture: ground collecting of wild cereals. Proc Natl Acad Sci 2015; 101:
2692-2695.
10. Miedaner T, Longin F. Unterschätzte Getreidearten Einkorn, Emmer, Dinkel& Co. AgriMedia GmbH & Co. KG, 2012; ISBN 978-3-86263-079-0.
11. Guandalini S. Historical perspective of celiac disease. In: Fasano A, Troncone R,
Branski D (eds): Frontiers in Celiac Disease. Pediatr Adolesc Med. Basel, Karger,
2008; 12: 1-11.
12. Rubio-Tapia A, Kyle RA, Kaplan EL, et al. Increased prevalence and mortality in
undiagnosed celiac disease. Gastroenterology 2009; 137: 88-93.
13. Ellis A, Linaker BD. Non-coeliac gluten sensitivity. Lancet 1978; 1: 1358-1359.
14. Wray D. Gluten-sensitive recurrent aphthous stomatitis. Digestive Diseases and
Sciences 1981; 26: 737-740.
15. Catassi C. Gluten sensitivity. Ann Nutr Metab 2015; 67 (suppl 2): 16-26.
16. The NPD Group/Dieting Monitor. 200 million restaurant visits include a glutenfree
order. www.ndp.com.
17. Gilissen LJWJ, Van der Meer IM, Smulders MJM. Reducing the incidence of
allergy and intolerance to cereals. J Cereal Sci 2014; 59: 337-353
18. Smulders MJM, Jouanin A, Schaart J, et al. Development of wheat varieties with
reduced contents of coeliac-immunogenic epitopes through conventional and GM
strategies. In: Koehler P (ed): Proceedings of the 28th Meeting, Working Group on
Prolamin Analysis and Toxicity. Deutsche Forschungsanstalt für
Lebensmittelchemie, Freising, 2015; pp. 47-56.
19. Kissing Kucek L, Veenstra LD, Ammuaycheewa P, et al. A grounded guide to
gluten: How modern genotypes and processing impact wheat sensitivity. Compr
Rev Food Sci Food Saf 2015; doi:10.1111/1541-4337.12129.
20. Van den Broeck HC, De Jong HC, Salentijn EMJ, et al. Presence of celiac disease
epitopes in modern and old hexaploid wheat varieties: wheat breeding may have
contributed to increase prevalence of celiac disease. Theor Appl Genet 2010; 121:
1527-1539.
21. Van den Broeck HC, Chen H, Lacaze X, et al. In search of tetraploid wheat
accessions reduced in celiac disease-related gluten epitopes. Mol Biosyst 2010; 6:
2206-2213.
22. Van den Broeck HC, Cordewener JHG, Nessen MA, et al. Label free targeted
detection and quantification of celiac disease immunogenic epitopes by mass
spectrometry. J Chromatography A 2015; 1391: 50-71.
23. Tanner GJ, Blundell MJ, Colgrave ML, et al. Creation of the first ultra-low gluten
barley (Hordeum vulgare L.) for coeliac and gluten-intolerant populations. Plant
Biotechnol J 2015; doi: 10.1111/pbi.12482
24. Van Herpen TWJM, Goryunova S, Van der Schoot J, et al. Alpha-gliadin genes
from the A, B and D genomes of wheat contain different sets of celiac disease
epitopes. BMC Genomics 7: 1.
25. Van den Broeck HC, Gilissen LJWJ, Smulders MJM, et al. Dough quality of bread
wheat lacking alpha-gliadins with celiac disease epitopes and addition of celiacsafe
avenins to improve dough quality. J Cereal Sci 2011; 53: 206-216.
26. Becker D, Wieser H, Koehler P, et al. Protein composition and techno-functional
properties of transgenic wheat with reduced alpha-gliadin content obtained by
RNA interference. J Appl Bot Food Qual 2012; 85: 23-33.
27. Gil-Humanes J, Pistón F, Tollefsen S, et al. Effective shutdown in the expression
of celiac disease-related wheat gliadin T-cell epitopes by RNA interference. Proc
Natl Acad Sci 2010; 107: 17023-17028.
28. Pistón f, Gil-Humanes J, Rodriguez-Quijano M, et al. Down-regulating gammagliadins
in bread wheat leads to non-specific increase in other gluten proteins and
has no major effect on dough gluten strength. PLoS ONE 2011; 6: e24754.
29. Gil-Humanes J, Pistón F, Giménez MJ, et al. The introgression of RNAi silencing
of gamma-gliadins into commercial lines of bread wheat changes the mixing and
technological properties of the dough. PLoS ONE 2012; 7: e45937.
30. Gil-Humanes J, Pistón F, Altamirano-Fortoul R, et al. Reduced-gliadin wheat
bread: an alternative to the gluten-free diet for consumers suffering gluten-related
pathologies. PLoS ONE 2014; 9: e90898.
31. Barro F, Iehisa JCM, Giménez MJ, et al. Targeting of prolamins by RNAi in bread
wheat: effectiveness of seven silencing-fragment combinations for obtaining lines
devoid of coeliac disease epitopes from highly immunogenic gliadins. Plant
Biotech J 2016; 14: 986-996.
32. Wen S, Wen N, Pang J, et al. Structural genes of wheat and barley 5-
methylcytosine DNA glycosylases and their potential applications for human
health. Proc Natl Acad Sci 2012; 109: 20543-20548.
33. Kasarda DD. Can an increase in celiac disease be attributed to an increase in the
gluten content of wheat as a consequence of wheat breeding? J Agric Food Chem
2013; 61: 1155-1159.
34. Gilissen LJWJ, Van den Broeck HC, Londono DM, et al. Food-related strategies
towards reduction of gluten intolerance and gluten sensitivity. In: Koehler P (ed):
Proceedings of the 25th Meeting, Working Group on Prolamin Analysis and
Toxicity. Deutsche Forschungsanstalt für Lebensmittelchemie, Freising, 2012; pp.
29-35.
35. Bassi S, Maningat CC, Chinaswamy R, et al. Alcohol-free wet extraction of gluten
dough into gliadin and glutenin. United States Patent, No 5,610,277.
36. Kratzer W, Kibele M, Porzner M, et al. Prevalence of celiac disease in Germany:
A prospective follow-up study. World J Gastroenterol 2013; 19: 2612-2620.
37. Mustalahti K, Catassi C, Reunanen A, et al. The prevalence of celiac disease in
Europe: Results of a centralized, international mass screening project. Ann Med
2010; 42: 587-595.
38. Laass MW, Schmitz R, Uhlig HH, et al. The prevalence of celiac disease in
children and adolescents in Germany. Dtsch Arztebl Int 2015; 112: 553-560.
39. Loponen J. Prolamin degradation in sourdoughs. Academic Dissertation. EKT
series 1372. University of Helsinki.
40. Greco L, Gobbetti M, Auricchio R, et al. Safety for patients with celiac disease of
baked goods made of wheat flour hydrolysed during food processing. Clin
Gastroenterol Hepatol 2011; 9: 24-29.
41. Stenman SM, Lindfors K, Venäläinen, et al. Degradation of coeliac diseaseinducing
rye secalin by germinating cereal enzymes: diminishing toxic effects in
intestinal elipthelial cells. Clin Exp Immunol 2010; 161: 242-249.
42. Schwalb T, Wieser H, Koehler P. Studies on the gluten-specific peptidase activity
of germinated grains from different cereal species and cultivars. Eur Food Res
Technol 2012; 235: 1161-1170.
43. Loponen J, Sontag-Strohm T, Venäläinen, et al. Prolamin hydrolysis in wheat
sourdoughs with differing proteolytic activities. J Agric Food Chem 2007; 55:
978-984.
44. Loponen J, Kanerva P, Zhang C, et al. Prolamin hydrolysis and pentosan
solubilization in germinated-rye sourdoughs determined by chromatographic and
immunological methods. J Agric Food Chem 2009; 57: 746-753.
45. Vader W, Kooy Y, Van Veelen P, et al. The gluten response in children with
celiac disease is directed towards multiple gliadin and glutenin peptides.
Gastroenterology 2002; 122: 1729-1737.
46. Camarca A, Anderson RP, Mamone G, et al. Intestinal T cell responses to gluten
peptides are largely heterogeneous: Implications for a peptide-based therapy in
celiac disease. J Immunol 2009; 182: 4158-4166.
47. Hollon J, Puppa EL, Greenwald B, et al. Effect of gliadin on permeability of
intestinal biopsy explants from celiac disease patients and patients with non-celiac
gluten sensitivity. Nutrients 2015; 7: 1565-1576.
48. Zuidmeer L, Goldhahn K, Rona RJ, et al. The prevalence of plant food allergies:
A systematic review. J Allergy Clin Immunol 2008; 121: 1210-1218.
49. Gilissen LJWJ, Van der Meer IM, Smulders MJM. Reducing the incidence of
allergy and intolerance to cereals. J Cereal Sci 2014; 59: 337-353.
50. Yazdanbakhsh M, Kremsner PG, Van Ree R. Allergy, parasites and the hygiene
hypothesis. Science 2002; 296: 490-494.
51. Rook GAW. Hygiene and other early childhood influences on the subsequent
function of the immune system. Dig Dis 2011; 29: 144-153.
52. Rook GAW. Hygiene hypothesis and autoimmune diseases. Clin Rev Allergy
Immunol 2012; 42: 5-15.
53. Sanz Y. Microbiome and gluten. Ann Nutr Metab 2015; 67 (suppl 2): 28-41.
54. Croese J, Giacomin P, Navarro S, et al. Experimental hookworm infection and
gluten microchallenge promote tolerance in celiac disease. J Allergy Clin Immunol
2015; 135: 508-516.
55. Giacomin P, Zakrzewski M, Croese J, et al. Experimental hookworm infection and
escalating gluten challenges are associated with increased microbial richness in
celiac disease 2015. Sci Rep 5: 13797; doi:10.1038/srer13797.
56. Hermsen LAH. The association between Helminth infections and atopic diseases.
In: Gao ZS, Shen HH, Zheng M, Frewer LJ, Gilissen LJWJ (eds):
Multidisciplinary approaches to allergies. Springer / Zhejiang University Press,
2012; pp. 561-473.
57. Wu H, Flint AJ, Qibin Q, et al. Association between dietary whole grain intake
and risk of mortality. JAMA Intern Med 2015; 173: 373-384.
58. Huang T, Xu A, Lee A, et al. Consumption of whole grains and cereal fibre and
total and cause-specific mortality: prospective analysis of 367,442 individuals.
BMC Medicine 2015; 13: 59 doi 10.1186/s12916-015-0294-7.
59. Davis W. Wheat Belly. Broadhead Ass., 2011; 292 pp.
60. Perlmutter D. Grain Brain. Hodder & Stoughton , 2014; 336 pp.
61. Brouns F, Gilissen L, Shewry P, et al. The war on wheat. How unsubstantiated
wheat and gluten related health concerns are damaging the cereal food sector and
comprising public health. The World of Food Ingredients 2015, September: 30-
31.
62. Shewry PR, Hey SJ. Do we need to worry about eating wheat? Nutrition Bulletin
2016; 41: 6-13.
63. Catassi C, Elli L, Bonaz B, et al. Diagnosis of non-celiac gluten sensitivity
(NGCS): The Salerno expert’s criteria. Nutrients 2015; 7: 4966-4977.
64. Brouns F, Gilissen L, Shewry P, et al. The cereal food supply chain is invited to
share responsibility for public health and help unravel wheat and gluten related
health concerns. Health Grain Forum 2015; 11 pp.
65. Gøtzsche P. Deadly medicines and organised crime: how big pharma has
corrupted healthcare. By P. C. Gøtzsche. London: Radcliffe Publishing, 2013; 310
pp. ISBN 978-184619-884-7
4.5 Ultra-low gluten barley
Gregory J. Tanner1, Malcolm J. Blundell1, Michelle L. Colgrave2, Crispin A. Howitt1
1 CSIRO Agriculture, Canberra, ACT 2601, Australia
2 CSIRO Agriculture, St Lucia, QLD 4067, Australia
Introduction
Coeliac disease (CD) is a condition that is estimated to affect about 1% of most
populations worldwide [1] and at present the only treatment is a lifelong gluten-free
diet with the exclusion of gluten-like proteins in wheat (gliadin and glutenins), barley
(hordeins), rye (secalins) and in some coeliacs, oats (avenins) [2]. Untreated coeliacs
face adverse health outcomes including low bone mineral density and increased
intestinal malignancy [3]. Gluten-free diets are traditionally more expensive, low in
fibre, high in fat and sugar, which in turn can lead to adverse health outcomes [4-6].
In addition to CD, a second condition known as non-celiac gluten sensitivity (NCGS)
is thought to affect up to 10% of the population in some countries [7,8]. Little is
known about NCGS, but it has been shown that for those who suffer NCGS a glutenfree
diet is beneficial.
Barley contains four classes of hordeins, the B-, C-, D-, and γ-hordeins. The B- and Chordeins
are the major classes representing over 90% of the hordeins [9,10]. The Bhordeins
and C-hordeins are encoded by the Hor2 and Hor1 loci, respectively, with
both loci located on the short arm of chromosome 1. In the 1970s, the search for high
lysine barleys at the Carlsberg Institute identified two mutants, Risø 56 and Risø 1508,
which had reduced hordein content. Risø 56 does not accumulate B-hordeins due to
the deletion of the Hor2 locus [9], while Risø 1508 does not accumulate C-hordeins
and this is the result of a mutation in the Lys3 locus on chromosome 5H [11]. The Dhordeins
are encoded by the Hor3 locus, which encodes a single 105 k protein [12],
located about 9 cM from the centromere on the long arm of chromosome 1H [13]. An
Ethiopian derived landrace, R118 [14], contains a single spontaneous mutation which
prevents accumulation of the D-hordeins. The γ-hordein family comprises two genes, γ-1 and γ-3 hordein at the Hor5 locus on the short arm of 1H which encode three γ-
hordein proteins (1, 2, and 3) [15,16]. The γ-hordein loci are very tightly linked to the
B-hordein locus at 0.2 cM [17,18].
We report the isolation of the first ultra-low gluten barley with hordein levels below
the Codex limit of 20 mg/kg for gluten in gluten-free food. In addition, the grain shape
and size have been improved and are similar to current malting lines. These new grains
have utility in the preparation of malt, food and beverages for those who suffer from
CD, wheat allergy and gluten intolerance.
Materials and methods
Barley lines cv. Sloop and Bomi (wild-types) were obtained from the Australian
Winter Cereals Collection (Tamworth, Australia). The single hordein-null lines, Risø 56 and Risø 1508 were obtained from the Nordic Germplasm Bank (Alnarp, Sweden).
A line which was null for D-hordein, Ethiopian R118 [14], was obtained from The
John Innes Centre Public Collections, Norwich. The Ethiopian R118 line was a landrace
observed to segregate for 2-row and 6-row phenotypes and for black pigmented
and green seeds. A two-rowed, green seeded line was selected and backcrossed to
Sloop to produce a BC2 D-hordein null line with 87.5% Sloop background.
Analytical methods
Plant analysis: Kernel weight, dimensions and screenings, a measure of seed size,
were determined using a SeedCountTM SC4 (Seed Count Australasia, Condell Park,
Australia). Total flour nitrogen was determined by the method of Dumas [19]. Starch, β-glucan, α-, and β-amylase were determined by the methods of McCleary [20-23].
Free sugar composition was by the anthrone method [24]. Fatty acids were extracted
and analysed according to the method of Zhou [25]. Free amino acids were determined
by the Australian Proteome Analysis Facility (Macquarie University, Sydney)
according to the method of Cohen [26] with norvaline (Sigma) as an internal standard
[27].
Protein Analysis: Protein extraction, one and two dimensional SDS PAGE and
ELISAs were carried out as described previously [28]. Mass spectrometry was
conducted as described previously [29-31].
Results and discussion
Creation and analysis of ULG 3.0
Risø 56 and Risø 1508 were intercrossed to produce a line that lacked B- and Chordeins
and was called ULG 2.0. The hordein concentration was lowered further by
crossing ULG 2.0 with the Ethiopian R118 line in a Sloop BC2 background to produce
ULG 3.0, which lacked B- C- and D-hordeins. Analysis of the hordein content in these
lines by ELISA (Tab. 1) revealed that it had been reduced to approximately 4 mg/kg in
ULG 3.0. 2D-PAGE, in-gel digestion and MS/MS analysis of ULG 3.0 confirmed that
the only detectable hordein in an alcohol soluble protein fraction from ULG 3.0 was γ-
3-hordein (Uniprot: P80198) (data not shown).
Seeds of both ULG 2.0 and 3.0 were shrunken due to the pleiotropic effect of the lys3a
gene derived from Risø 1508 [32] and had high levels of screenings. When grown in
the field at Ginninderra Experiment Station, ACT, seed weight of ULG 2.0 was 33.5± 0.40 mg/ kernel, while ULG 3.0 had slightly larger seeds 39.2 ± 0.31 probably due to
the contribution of cv. Sloop germplasm. The screenings also decreased dramatically
from ULG 2.0 (96.2 ± 1.2% of grains <2.8 mm) to ULG 3.0 (41.3 ± 3.7% <2.8 mm).
Table 1. Hordein content of ULG lines as measured by ELISA, values are mean ± SE,
n=3.
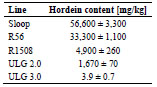
Improving seed size, development of ULG 3.2
ULG 2.0 was crossed to cv. Sloop, Yagan and Baudin. F2 hordein double-null lines
were identified in the three backgrounds 2S (Sloop), 2Y (Yagan) and 2B (Baudin)
respectively, each containing 50% of the respective parental germplasm. These
hordein double-null lines were crossed with a ULG 3.0 line named T2 and F2 hordein
tri-nulls identified in the three backgrounds T2S, T2Y and T2B respectively. The
hordein tri-null lines were intercrossed in pairs in both directions e.g. T2S x T2Y and
T2Y x T2S, to form F1 lines, SY and YS, respectively, to create biparental lines, all
with hordein tri-null phenotypes. The F1 lines were either selfed to create F2 biparental
families or intercrossed and selfed to create segregating quad-parental
families all with a hordein tri-null phenotype.
Through a field selection process over two generations the best 20 lines were
identified. Progeny of these lines were carried through a further three generations of
field selection to identify agronomically acceptable lines that produced seeds that were
larger, with short, well-filled heads. The best two lines, 43.2 and 124.1, were selected
and fixed by three rounds of single-seed descent. These lines had the lowest hordein
levels, and improved seed weight (>47.2 mg), and improved screenings near that
expected for commercial malting barley (3-14%).
Proximal analysis of ULG 2.0, 3.0, and 3.2
Starch, monosaccharides, β-glucan, free amino acids, and fatty acid content were also
determined to establish whether removal of seed hordeins, a significant seed sink,
impacted other components of the grain (Tab. 2).
The starch content of ULG 3.0 was lower than the controls Sloop and Baudin, while
the two ULG 3.2 lines 043.1 and 124.1 had starch contents similar to the controls.
There was no consistent trend in monosaccharide content between the lines with 043.2
and 124.1 having similar levels to that seen in Baudin. The protein content of ULG 3.0
was higher than that seen in the control lines, while the 043.2 and 124.1 had a lower
protein content than the controls. Interestingly, the β-glucan content of the ULG 3.0
lines was significantly lower than that of the controls, with 043.2 and 124.1 having a lower β-glucan content than ULG 3.0. The cause of this is unknown at present. The
total free amino acid content in the ULG 3.0 lines was up to 15-fold higher than the
levels in the controls and is likely to be a direct result of the absence of the hordeins
and the inability of the lines to incorporate proline and glutamine, which are present in
high concentrations in the hordein proteins, into other proteins in the absence of the
hordeins.
Amylase activity
The level of α-amylase of the ULG 3.2 lines was not significantly different from the
malting lines cv. Sloop, Baudin, Commander or Hindmarsh. Conversely, the level of β-amylase in the ULG 3.2 lines was reduced by approximately 10-fold compared to
the malting lines above (data not shown).
Table 2. Proximal analysis of grain components from the ULG lines.
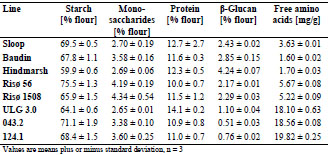
Conclusions
We report the selection and breeding of the first ultra-low gluten barley. The hordein
level was extremely low, to the point where it was difficult to measure even with
sensitive mass spectrometry or ELISA. The grain had a normal appearance and
malting and brewing properties sufficient to make a useful malting grain. This grain
has application in the preparation of food and beverages for coeliacs and gluten
intolerants.
Acknowledgements
We thank the Grains Research and Development Corporation (GRDC) of Australia for
providing financial assistance for this work. Thanks also to Russell Heywood (CSIRO
Agriculture, Canberra) who carried out the field work and to James Tanner who
harvested and analysed the single seed descent of three generations of ULG 3.2 plants.
References
1. Fasano A, Berti I, Gerarduzzi T, et al. Prevalence of celiac disease in at-risk and
not-at-risk groups in the United States - a large multicenter study. Arch Intern Med
2003; 163: 286-292.
2. Hardy MY, Tye-Din JA, Stewart JA, et al. Ingestion of oats and barley in patients
with celiac disease mobilizes cross-reactive T cells activated by avenin peptides
and immuno-dominant hordein peptides. J Autoim 2014; 56: 56-65.
3. Green PHR, Fleischauer AT, Bhagat G, et al. Risk of malignancy in patients with
celiac disease. Am J Med 2003; 115: 191-195.
4. Lee, A.R., D.L. Ng, J. Zivin, et al., Economic burden of a gluten-free diet. J Hum
Nutr Diet, 2007. 20: 423-430.
5. Wild D, Robins GG, Burley VJ, et al. Evidence of high sugar intake, and low fibre
and mineral intake, in the gluten-free diet. Aliment Pharm Therap 2010; 32: 573-
581.
6. Ohlund K, Olsson C, Hernell O, et al. Dietary shortcomings in children on a
gluten-free diet. J Human Nutr Diet 2010; 23: 294-300.
7. Aziz I, Lewis NR, Hadjivassiliou M, et al. A UK study assessing the population
prevalence of self-reported gluten sensitivity and referral characteristics to
secondary care. Eur J Gastroenterol Hepatol 2014; 26: 33-9.
8. Golley S, Corsini N, Topping D, et al. Motivations for avoiding wheat
consumption in Australia: results from a population survey. Pub Health Nutrit
2015; 18: 490-9.
9. Kreis M, Shewry PR, Forde BG, et al. Molecular analysis of a mutation conferring
the high-lysine phenotype on the grain of barley (Hordeum vulgare). Cell 1983;
34: p161-167.
10. Shewry PR, Bunce NAC, Kreis M, et al. Polymorphism at the Hor 1 locus of
barley (Hordeum vulgare L.). Biochem Genet 1985; 23: 391-404.
11. Karlsson K. Linkage studies on a gene for high lysine content in Riso barley
mutant 1508. Barely Genetics Newsletter 1977; 7: 40-43.
12. Gu YQ, Anderson OD, Londeore CF, et al. Structural organization of the barley
D-hordein locus in comparison with its orthologous regions of wheat genomes.
Genome 2003; 46: 1084-1097.
13. Shewry PR, Finch RA, Parmar S, et al. Chromosomal location of Hor 3, a new
locus governing storage proteins in barley. Heredity 1983; 50: p179-189.
14. Brennan CS, Smith DB, Harris N, et al. The production and characterisation of
Hor 3 null lines of barley provides new information on the relationship of D
hordein to malting performance. J Cereal Sci 1998; 28: 291-299.
15. Shewry PR, Kreis M, Parmar S, et al. Identification of gamma-type hordeins in
barley. FEBS Letters 1985; 190: 61-64.
16. Cameron-Mills V, Brandt A. A gamma-hordein gene. Plant Mol Biol 1988; 11:
p449-461.
17. Shewry PR, Parmar S, Franklin J, et al. Mapping and biochemical analysis of Hor
4 (Hrd-G), a 2nd locus encoding B-hordein seed proteins in barley (Hordeum
vulgare L.). Genet Res 1988; 51: 5-12.
18. Shewry PR, Parmar S. The HRDF (Hor 5) locus -type hordeins. Barley Genetics
Newsletter 1987; 17: 32-34.
19. Shea F, Watts CE. Dumas method for organic nitrogen. Ind Eng Chem Anal Ed
1939; 11: 333-334.
20. McCleary BV, Codd R. Measurement of (1-3) (1-4)-β-D-glucan in barley and
oats: a streamlined enzymic procedure. J Sci Food Ag 1991; 55: 303-312.
21. McCleary BV, Gibson TS, Mugford DC. Measurement of total starch in cereal
products by amyloglucosidase - α-amylase method: Collaborative study. J AOAC
Int 1997; 80: 571-579.
22. McCleary BV, McNally M, Monaghan D, et al. Measurement of α-amylase
activity in white wheat flour, milled malt, and microbial enzyme preparations
using the Ceralpha assay: collaborative study. J AOAC Int 2002; 85: 1096-1102.
23. McCleary BV, Codd R. Measurement of ß-amylase in cereal flours and
commercial enzyme preparations. J Cereal Sci 1989; 9: 17-33.
24. Yemm EW, Willis AJ. The estimation of carbohydrates in plant extracts by
anthrone. Biochem J 1954; 57: 508-514.
25. Zhou X-R, Green AG, Singh SP. Caenorhabditis elegans 12-desaturase FAT-2 is
a bifundDesaturase able to desaturate a diverse range of fatty acid substrates at the
12 and 15 positions. J Biol Chem 2011; 286: 43644-43650.
26. Cohen SA. Amino acid analysis using precolumn derivatisation with 6-
aminoquinolyl-N-hydroxysuccinimidyl carbamate. In: Methods in Molecular
Biology., C. Cooper, N. Packer, and K. Williams, Editors. 2001; Humana Press.:
Totowa, NJ, USA. 39-47.
27. Boeuf P, Aitken EH, Chandrasiri U, et al. Plasmodium falciparum malaria elicits
inflammatory responses that dysregulate placental amino acid transport. PLoS
Pathogens 2013; 9: e1003153.
28. Tanner GJ, Colgrave ML, Blundell MJ, et al. Measuring hordein (gluten) in beer– A comparison of ELISA and mass spectrometry. PLoS ONE 2013; 8(2): e56452.
29. Colgrave ML, Goswami H, Blundell M, et al. Using mass spectrometry to detect
hydrolysed gluten in beer that is responsible for false negatives by ELISA. J
Chromatogr A 2014; 1370: 105-114.
30. Colgrave ML, Goswami H, Byrne K, et al. Proteomic profiling of 16 cereal grains
and the application of targeted proteomics to detect wheat contamination. J
Proteome Res 2015; 14: 2659-2668.
31. Colgrave ML, Goswami H, Howitt CA, et al. What is in a beer? Proteomic
characterization and relative quantification of hordein (gluten) in beer. J Proteome
Res 2012; 11: 386-396.
32. Gabert VM, Brunsgaard G, Eggum BO, et al. Protein quality and digestibility of
new high-lysine barley varieties in growing rats. Plant Foods Hum Nutr 1995; 48:
169-79.
4.6 Developing a hordein standard for barley-containing
products
Xin Huang1, Päivi Kanerva2, Tuula Sontag-Strohm1
1 University of Helsinki, Helsinki, Finland
2 Fazer Mixes & Mills, Lahti, Finland
Introduction
The use of a gliadin reference material for barley-containing products in the prolamin
quantification by R5 ELISA causes overestimation [1,2]. The difference in prolamin
composition between wheat and barley, and the different reactivity of each prolamin
group with the antibody are the main reasons. To obtain an accurate value, a more
specific reference material is needed when measuring 1) prolamin content in glutenfree
barley starch; 2) prolamin content in gluten-free beer; 3) barley contamination in
oat products. In this study, we investigated barley cultivars from several regions of
origin, and analysed their behaviour in R5 ELISA quantification and their prolamin
composition.
Materials and methods
A collection of common barley cultivars from Nordic countries, central Europe and
North America was kindly provided by Boreal Plant Breeding Ltd., Finland. After
water and 0.5 mol/L NaCl extraction of milled grains, the prolamins were extracted by
40% (v+v) 1-propanol with 1% (w+v) dithiothreitol (DTT), 30 min at 50 °C. To study
the prolamin composition, prolamins of barley cultivars were separated by sodium
dodecylsulphate-polyacrylamide gel electrophoresis (SDS-PAGE; 10% Bis-Tris,
NuPage, Invitrogen). The gel was stained by Coomassie Brilliant Blue R-250 for 1 h,
and destained for 2 h with 7.5% (v+v) acetic acid and 10% (v+v) ethanol. The gel was
visualised by AlphaImager HP system (ProteinSimple, California, USA), prolamin
bands were detected and the density was calculated.
The milled grains were also extracted by RIDA Extraction Solution (colourless) (RBiopharm,
Darmstadt, Germany). After optimised dilution, the prolamins were
measured by sandwich R5 ELISA (R7001 RIDASCREEN Gliadin, R-Biopharm). In
order to get the actual protein content, the RIDA extraction solutes were dialysed
against water to remove the reagents prior to analysis, then dried and analysed by the
Dumas method (nitrogen content × 6.25).
Results and discussion
From SDS-PAGE, the hordein pattern of cultivars can be divided into three groups by
size, D-hordein, C-hordein, and B + -hordein (Fig. 1). When protein bands were taken
for gel densitometry, we could compare the proportion of each hordein group among
cultivars. The D-hordein size and proportion was very similar among different
cultivars, around 10% of total hordein. However, C-hordein composition was different.
For example, cultivar 79, 80, and Vilde had more bands than cultivar 88, 89, and
Harbinger. The reported C-hordein composition was 10-20% of total hordein [3].
However we found that some cultivars had a C-hordein content around 30% of total
hordein (Tab. 1, cultivar 51 and Scarlett). The R5 antibody recognized -type of
prolamin; in the case of barley, the R5 antibody recognized C-hordein better than other
hordein groups.
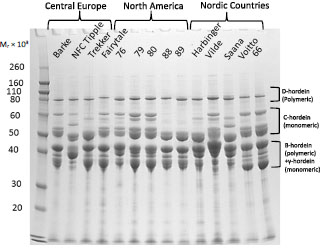
Figure 1. SDS-PAGE of barley hordein of selected barley cultivars originating from
Central Europe, North America, and Nordic countries
Our hypothesis was that the high proportion of C-hordein was the reason for
overestimation of barley prolamin quantification by the R5 antibody. When the Chordein
proportion was around 15% of total hordein, like -gliadin 10-20% of total
gliadin in wheat (Shewry and Tatham, 1990), the response matched with the gliadin standard (cultivar KWS Asta and Overture in Fig. 2). However, when looking at the
distance between actual prolamin concentration and gliadin standard curve, the
distance was greater at higher C-hordein proportion, which means more
overestimation. The primary structure of C-hordein, composed of repetitive sequences
of PQQPFPQQ, is one plausible cause of this overestimation. The R5 epitope QQPFP
appeared 13 times in C-hordein, but only 5 times in B1-hordein and 3 times in B3-
hordein. Other epitopes QQPYP and QLPFP, which are also recognised by the R5
antibody, appear once in C-hordein. When measuring pure C-hordein with R5 ELISA,
the concentration was 15-20 times overestimated in the sandwich ELISA with gliadin
standard, and 23-43 times overestimated in the competitive ELISA with hydrolysed
prolamin standard (Huang et al., 2016). -Gliadin also contains a high number of
epitope repeats (QQPFP×14, QLPFP×1, QQPYP×4, QQSFP×3), but the -gliadin
proportion of whole gliadin does not vary that much among cultivars.
Table 1. Hordein composition of selected barley cultivars.
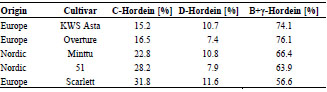
We propose a hordein reference material from only one barley cultivar, instead of a
combination of many cultivars. This cultivar should have an average C-hordein
proportion, based on cultivars cultivated in the region. For example, the barley
cultivars in Finland seem to have a higher C-hordein proportion than the ones in
Central Europe, probably due to the climate influence. The one cultivar-based hordein
reference material can be used to measure the prolamin content in barley starch with
sandwich ELISA. The hydrolysed hordein can serve as a reference material in the
competitive ELISA for measuring the prolamin content of barley-based beer. Another
application of the hordein standard is to measure the barley contamination in oat
products. However, this situation is more complicated and needs to be combined with
other techniques, like mass spectrometry, to identify whether the contamination comes
from wheat or barley beforehand.
Conclusions
The C-hordein proportion in barley cultivars varies much more than -gliadin in
wheat. In general, the relative proportion of -type prolamin is higher in barley than in
wheat. It is one plausible reason for overestimation of gluten in barley-containing
products if a (wheat) gliadin standard is used in the gluten assays. We propose a hordein reference material from one cultivar, which contains an average C-hordein
proportion of total hordein in cultivars used in one region. Each region may have their
own reference material, based on their most common cultivars.
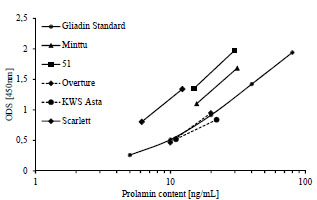
Figure 2. The RIDA extraction solutes response in the R5 sandwich ELISA. The
prolamins were extracted by RIDA extraction solution, the actual prolamin content
was measured by Dumas nitrogen content × 6.25
References
1. Kanerva P, Sontag-Strohm T, Ryöppy P, et al. Analysis of barley contamination in
oats using R5 and omega-gliadin antibodies. J Cereal Sci 2006; 44: 347-352.
2. Tanner GJ, Blundell, MJ, Colgrave ML, et al. Quantification of hordeins by
ELISA: The correct standard marks a magnitude of difference. PLOS One 2013;
(8), e56456.
3. Tatham AS, Shewry PR. The S-poor prolamins of wheat, barley and rye. J Cereal
Sci 1995; 22: 1-16.
4. Shewry PR, Tatham AS. The prolamin storage proteins of cereal seeds: structure
and evolution. J Biochem 1990; 267: 1-12.
5. Huang X, Kanerva P, Salovaara H, et al. Degradation of C-hordein by metalcatalysed
oxidation. Food Chemistry 2016; 196: 1256-1263.
5. Clinical research reports
5.1 Evaluation of diet composition and food habits in the
Spanish coeliac population
Idoia Larretxi, Itziar Churruca, Jonatan Miranda, Arrate Lasa, María Á. Bustamante, Edurne Simon
University of the Basque Country, Gluten Analysis Laboratory, Vitoria-Gasteiz, Spain
Introduction
The only treatment for coeliac disease (CD) consists of following a gluten-free (GF)
diet and the ingestion of small amounts of gluten can cause major disruptions in gluten
intolerants. Thus, an increasing interest in GF foodstuffs has arisen in recent years and
the GF product market has become one of the most profitable markets in the field of
food and beverages.
Although the target of this market has focused on the absence of gluten in GF foods,
the nutritional quality of GF foodstuffs is an important aspect to consider [1]. Some
authors have demonstrated that 20-38% of coeliac patients [2,3] show some nutritional
deficiencies due to an inadequate intake of calories/protein [4], dietary fibre [5-7] or
various micronutrients [7-9].
The correct GF diet should fulfil all energy and nutrient requirements and prevent
individuals from any nutritional deficiency. Sometimes, following a GF diet implies an
excessive restriction of cereal consumption in order to avoid gluten intake. This
circumstance may lead to a low carbohydrate intake with an excess of fat and protein.
Indeed, some studies highlight excessive saturated fats in GF products [5,10]. With
regard to micronutrient intake, it has been shown that following a GF diet could also
result in low intake of fibre and some micronutrients such as iron, folate, niacin, and
zinc. Other authors also indicate that consumption of refined grains, which are used in
the manufacture of processed GF products, entails a lower intake of B group vitamins,
D vitamin and calcium [11].
In view of the above, the aim of the present work was to evaluate the possible
consequences of following a GF diet in the nutritional requirements of coeliac patients.
For this purpose, we evaluated the diet composition of Spanish coeliacs and measured
their compliance with a balanced and healthy diet. Furthermore, we studied the
nutritional composition of GF-rendered foodstuffs to highlight any difference with
equivalent foods containing gluten.
Material and methods
Nutritional composition of GF-rendered foodstuffs and comparison to equivalent
products with gluten
Nutritional information available on labels was analysed from GF-rendered foods (n =
206) as well as that of equivalent products containing gluten (n = 289). Foodstuffs
were classified into different groups using the reference given by Gibert et al. [12].
Information about energy content, total and simple carbohydrates, proteins, total and
saturated lipids, fibre, sodium, salt and cholesterol was collected. These data were
included in a spreadsheet, and the nutritional composition of GF-rendered foods was
compared to that of products containing gluten.
Dietary Assessment
Data from a female coeliac cohort recruited in 2011 from 3 regions in the north of
Spain (Alava, Gipuzkoa, and Bizkaia) were used in the present study (n = 54, mean
age ± SD: 34 ± 13). All participants had been compliant with the GF diet for at least
one year, as previously described [13].
24-hour food recall for 3 days (two weekdays and one weekend day) and a food
frequency questionnaire were collected for each woman. Food servings and amounts
were determined using photographs and nutrient intake was calculated by a
computerized nutrition program system (AyS, Software, Tandem Innova, Inc.).
The nutrient content of the specific GF products destined for coeliac people was
collected from the manufacturers and added into the food composition database before
calculations. As GF product labels did not indicate micronutrient contents (vitamin and
minerals), an estimation with homologous products containing gluten was made [14].
Energy, nutrient and food intake of coeliac women was compared to that of a reference
Spanish women population (data from ENIDE study) [15].
Statistical analysis
Statistical analyses were performed by using the IBM SPSS statistical program 19
(IBM Inc., Armonk, NY, USA). The results for continuous variables are given as the
arithmetic mean ± SD and the range, whereas results for non-continuous variables are
given as the frequency and the percentage. Statistical analyses were performed with
Student’s t test. P values <0.05 were accepted as significant.
Results and discussion
Nutritional composition of GF-rendered foodstuffs and comparison to equivalent
products with gluten
When the composition of GF-rendered foodstuffs was compared to that of glutencontaining
products significant differences were found (Fig. 1).
The group of GF breads and pasta had almost a third less protein than the equivalent
gluten-containing foods (p<0.001). This fact has also been recently reported by others
[16]. Besides, and in good accordance with Segura et al. [1], GF breads provided twice
as much fat (p=0.001), mainly in the form of saturated fat. Additionally, the pasta
group in GF products had declared more sodium and less fibre, as observed previously
by our group in the case of cereal bars or flour [14].
Some of these nutritional differences could be based on the list of ingredients used. It
is common for starches, hydrocolloids, gums, enzymes and other protein sources
(soybean, amaranth, quinoa, etc.) to be used to produce GF products in order to
improve their viscoelasticity and some other nutritional aspects [17,18]. The
ingredients added for the optimization of texture and/or palatability could explain the
higher fat intake, whereas the lower fibre intake might be due to qualitative or
quantitative differences among bread and cereals in a GF diet.
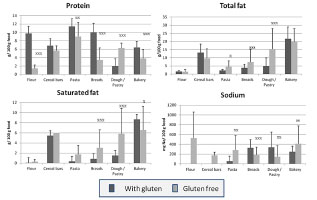
Figure 1. Protein, total and saturated fat, and sodium label content per 100 g of
product in gluten-containing and GF-rendered foodstuffs classified by food groups.
Results are given as means with the positive error, shown by vertical bars. (x p<0.05,
xx p<0.01; xxx p<0.001)
Dietary Assessment
The average energy intake of coeliac women was in good accordance with their
estimated energy expenditure, but was slightly lower than the dietary intake
recommendations [19], which is similar to that observed in the Spanish female
population in ENIDE studies [15]. Furthermore, when energy distribution was
analysed, no differences were found between both groups (Tab. 1).
Excessive protein intake (17.3% of total energy intake) in coeliac women was due to a
high consumption of animal protein (69% of total protein). With reference to this, an
excessive consumption of meat was seen in more than three quarters of participants.
Carbohydrate (CHO) consumption (41.9% of total energy intake) was enough to cover
the minimum established as Recommended Dietary Allowance (RDA) (130 g/day) by
the Institute of Medicine of The National Academies (IoM) [20], but it was far from
being the 45 to 60% of total energy proposed as the dietary reference value (DRV).
Nevertheless, it should be pointed out that other research conducted with coeliac
women members of the German Coeliac Society showed more adherence to the
carbohydrate consumption (45.9% of total energy) [21].
With regard to lipid intake, the percentage of this macronutrient markedly exceeded
the recommendations (Tab. 1), established as 30 to 35% of total energy. When fibre
consumption was analysed, the coeliac group consumed a significantly lower daily
amount than Spanish women from the ENIDE survey (16.4 ± 5.6 vs 18.9 ± 10.1;
p<0.001). According to our data, coeliac women older than 45 years (n = 13)
demonstrated better adherence to the Mediterranean diet and this was reflected in fibre
consumption. This group consumed on average 19.0 ± 5.1 g/day while the registered
intake in young adult coeliac women (younger than 45 years, n = 41) was 15.6 ± 5.5
g/day (p=0.005).
Table 1. Energy and nutrient distribution in coeliac women and Spanish women
(control women, ENIDE study).
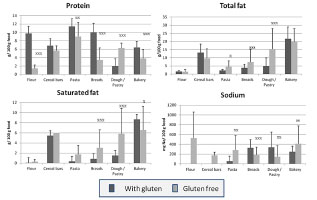
As we previously reported [13], food consumption frequency data (Fig. 2) showed that
cereal intake was very low in nearly all women. In fact, 48% consumed fewer than two
servings per day and only 7% of coeliac women fulfilled cereal recommendations (4-6
servings per day). With regard to the source of cereals in the diet, most of the cereal derivatives were naturally GF grains and not specifically GF-rendered products (3% of
total energy intake).
The majority of participants, 70%, did not reach vegetable consumption
recommendations (10 portions/week) [22], and one third of coeliac women did not
consume the minimum recommended fruit quantity, two servings daily.
In connection with animal source foodstuffs, it is important to mention that
consumption of meat and meat products was excessive in a large population of
coeliacs (>75%), and dairy servings were also fulfilled in a large percentage (87%).
Regarding the consumption of legumes, 65% of coeliac women usually included two
or more portions per week. When comparing these data with the Spanish general
population [23], a great difference between the groups was observed (19.3 g/d vs 11.9
g/d). The strategy followed by coeliac people consisted of avoiding gluten in the diet,
diminishing cereal intake and promoting the consumption of legumes instead of
cereals [24].
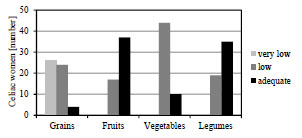
Figure 2. Food frequency consumption (classified as very low, low or adequate) of
coeliac women, expressed as number of women, according to Spanish Society of
Community Nutrition (SENC) recommendations (grains: very low: <2 servings/day;
low: 2-4 servings/day; adequate: >4 servings/day; fruits and vegetables: low: <2
servings of each per day; adequate: >2 servings of each per day; legumes: low: <2
servings/week; adequate: >2 servings/week)
Conclusions
Considering the dietary assessment of coeliac adult women, it could be postulated that
coeliac women and Spanish women -control women- follow similar trends as a whole,
with an imbalanced distribution of macronutrients and an inadequate consumption of
certain dietary components. Food frequency analysis suggests that some specificnutritional education might be provided to coeliac people in order to improve eating
habits and the nutritional status among adult coeliac women.
Furthermore, according to our results, there are marked differences between following
a diet with GF products and a diet with gluten-containing products.
Taking into account the composition of GF-rendered foods, it might be thought that
new ingredients based on vegetable sources would be helpful in improving these
nutritional values. Additionally it is possible to draw the conclusion that in order to
avoid wrong nutritional estimations, the different nutritional composition of products
rendered GF demands specific diet design software containing a database of GF
product compositions. As stated in the material and methods section, at the time when
the present research was carried out, no software contained such a database.
In order to fill this gap, our group has recently developed appropriate software for the
nutritional evaluation of a GF diet. This tool is available on www.ehu.eus/es/web/
laboratorio_gluten/softwareak.
References
1. Segura ME, Rosell CM. Chemical composition and starch digestibility of different
gluten-free breads. Plant Foods Hum Nutr 2011; 66: 224-230.
2. Kinsey L, Burden ST, Bannerman E. A dietary survey to determine if patients with
coeliac disease are meeting current healthy eating guidelines and how their diet
compares to that of the British general population. Eur J Clin Nutr 2008; 62:1333-
1342.
3. Saturni L, Ferretti G, Bacchetti T. The gluten-free diet: safety and nutritional
quality, Nutrients 2010; 2: 16-34.
4. Bardella MT, Fredella C, Prampolini L, et al. Body composition and dietary
intakes in adult celiac disease patients consuming a strict gluten-free diet. Am J
Clin Nutr 2000; 72: 937-939.
5. Thompson T, Dennis M, Higgins LA, et al. Gluten-free diet survey: are Americans
with coeliac disease consuming recommended amounts of fibre, iron, calcium and
grain foods?. J Hum Nutr Diet 2005; 18: 163-169.
6. Thompson T. Folate, iron, and dietary fiber contents of the gluten-free diet. J Am
Diet Assoc 2000; 100: 1389-1396.
7. Barton SH, Kelly DG, Murray JA. Nutritional deficiencies in celiac disease.
Gastroenterol Clin North Am 2007; 36: 93-108.
8. Baydoun A, Maakaron JE, Halawi H, et al. Hematological manifestations of celiac
disease. Scand J Gastroenterol 2012; 47: 1401-1411.
9. Tikkakoski S, Savilahti E, Kolho KL. Undiagnosed coeliac disease and nutritional
deficiencies in adults screened in primary health care. Scand J Gastroenterol
2007; 42: 60-65.
10. Hopman EG, le Cessie S, von Blomberg BM, et al. Nutritional management of the
gluten-free diet in young people with celiac disease in The Netherlands. J Pediatr
Gastroenterol Nutr 2006; 43: 102-108.
11. American Dietetic Association (ADA). Celiac disease (CD). Evidence based
nutrition practice guideline. American Dietetic Association, Chicago, 2009;
Various p.
12. Gibert A, Kruizinga AG, Neuhold S, et al. Might gluten traces in wheat substitutes
pose a risk in patients with celiac disease? A population-based probabilistic
approach to risk estimation. Am J Clin Nutr 2013; 97: 109-116.
13. Churruca I, Miranda J, Lasa A, et al. Analysis of body composition and food
habits of Spanish celiac women. Nutrients 2015; 7: 5515-5531.
14. Miranda J, Lasa A, Bustamante MA, et al. Nutritional differences between a
gluten-free diet and a diet containing equivalent products with gluten. Plant Foods
Hum Nutr 2014; 69: 182-187.
15. Nutritional Assessment Studies: Spanish Population Dietary Intakes.
National Health Survey. ENIDE. Available online: http://aesan.msssi.gob.es/
AESAN/web/evaluacion_riesgos/seccion/estudios_evaluacion_nutricional.shtml
(accessed on 24 October 2015).
16. Wu JH, Neal B, Trevena H, et al. Are gluten-free foods healthier than non-glutenfree
foods? An evaluation of supermarket products in Australia. Br J Nutr 2015;
114: 448-454.
17. Mäkinen OE, Zannini E, Arendt EK. Germination of oat and quinoa and
evaluation of the malts as gluten free baking ingredients. Plant Foods Hum Nutr
2013; 68: 90-95.
18. Cornejo F, Caceres PJ, Martínez-Villaluenga C, et al. Effects of germination on
the nutritive value and bioactive compounds of brown rice breads. Food Chem
2015; 15: 298-304.
19. Federation of Spanish Societies of Nutrition and Dietetics (FESNAD). Dietary
reference intakes (DRI) for spanish population, 2010. Act. Diet. 2010; 14: 196-
197.
20. Ross C, Taylor C, Yaktine A, et al. Dietary Reference Intakes for Calcium and
Vitamin D. The National Academies Press, Washington, USA, 2011; 1132p.
21. Martin J, Geisel T, Maresch C, et al. Inadequate nutrient intake in patients with
celiac disease: Results from a German dietary survey. Digestion 2013; 87, 240– 246.
22. Aranceta J, Serra-Majem L. Dietary guidelines for the Spanish population. Public.
Health Nutr 2001; 4: 1403-1408.
23. Varela-Moreiras G, Avila JM, Cuadrado C, et al. Evaluation of food consumption
and dietary patterns in Spain by the Food Consumption Survey: updated
information. Eur J Clin Nutr 2010; 64 Suppl 3: S37-43.
24. Mlynekov Z, Chrenkov M, Formelov Z. Cereals and Legumes in Nutrition of
People with Celiac Disease. Int J Celiac Dis 2014; 2: 105-109.
5.2 Innate immune signalling pathways induced by
gliadin peptide p31-43
Maria Florencia Gomez Castro1, Paula Carasi2, Allan Mowat3, Elena F. Verdu4,
Fernando Chirdo1
1 Instituto de Estudios Inmunológicos y Fisiopatológicos (IIFP-CONICET/UNLP),
Buenos Aires, Argentina
2 Cátedra de Microbiología General, Facultad de Ciencias Exactas (UNLP), Buenos
Aires, Argentina
3 Centre for Immunobiology, Institute of Infection, Immunity and Inflammation,
University of Glasgow, Glasgow G12 8TA, Scotland, UK
4 Farncombe Institute, McMaster University, Hamilton, Canada
Introduction
Several studies have shown that a set of gluten-derived peptides are immunogenic and
induce a potent gluten-specific CD4+ T-cell response in the small intestinal mucosa in
CD patients [1]. Particularly, transglutaminase 2 converts native into deamidated
gluten peptides, which bind to HLA-DQ2 and -DQ8 molecules with higher affinity,
thus enhancing T-cell activation [2]. On the other hand, some gluten peptides may
mediate non-HLA related effects, which could lead to innate immune activation [3].
However the signalling pathways of innate immune activation by gluten peptides
remain unclear.
The α-gliadin peptide, p31-43, which does not bind to HLA-DQ2/DQ8, has been used
as a peptide model to study the innate responses that may be relevant in CD [3]. The
described effects of p31-43 include apoptosis induction [4], upregulation of stressinducible
MHC class I polypeptide-related molecules (MICA) [5], and inhibition of
epidermal growth factor receptor (EGFR) endocytic pathways [6]. P31-43 also delays
traffic of EGF-carrying vesicles and late endosomes maturation in epithelial cells [7].
Most of the knowledge on the effects of p31-43 arises from in vitro studies using cell
lines, duodenal biopsies or cells isolated from human samples [3]. To date, there is no
evidence that p31-43 exerts any innate immune activation in vivo. Previous studies
developed by our group have shown that p31-43 triggers enteropathy in a murine
model that develops reduced villus height/crypt depth (V/C) ratio, infiltration of
intraepithelial lymphocytes (IELs), increased cell death in the lamina propria and
proliferative activity on the crypts [10].
Aims
Thus, the aim of this study was to evaluate the in vivo signalling pathways involved in
innate immune activation by p31-43.
Materials and methods
Six to eight week old C57BL/6, MyD88-/-, IFNR-/- and C3H-HeJ mice received an
intraluminal injection, by a microsurgical procedure, of p31-43 (100 μL, 100 μg/mL,
10 μg per mouse) or phosphate-buffered saline (PBS), following a procedure
previously described [10, 11]. Mice were sacrificed 16 h post-surgery. Small intestinal
samples were collected and fixed with formalin. 5 μm Paraffin-embedded sections
were obtained, re-hydrated and stained with H&E.
Histological changes were evaluated using the V/C ratio and the number of IELs.
Villus and crypt length ratio were measured on H&E stained intestinal sections.
Pictures were obtained with a Nikon Eclipse Microscope and morphometric analysis
was performed using a properly calibrated, image processing program (ImageJ). IELs
were counted on 10 randomly chosen villus tips and results were expressed as IEL/100
enterocytes. For the statistical analysis an unpaired t-test and ANOVA were used.
Results and discussion
Inflammatory changes induced by p31-43 are myeloid differentiation primary
response gene 88- (MyD88) dependent
In vitro studies have demonstrated that induction of proinflammatory cytokines and
increased intestinal permeability driven by p31-43 are MyD88-dependent [12]. After
intraluminal administration of p31-43 in C57BL6 mice, we observed intestinal
damage, characterised by reduction in V/C ratio and increment in the numbers of IELs
(Fig. 1A). We then tested whether MyD88, a key adaptor molecule in the TLR/IL-1R
signalling pathways, is also involved in the effect induced by p31-43 in vivo. V/C ratio
and number of IELs in MyD88 KO mice treated with p31-43 were similar compared
with PBS-controls (Fig. 1B). Thus, MyD88 is critical for the in vivo development of
small bowel inflammation in this model.
p31-43 effects are toll-like receptor 4- (TLR4) independent
TLR4-defective C3H/HeJ mice were used to evaluate whether TLR4 signalling
pathway is involved in p31-43 induced effects. The morphometric analysis
demonstrated reduction of V/C ratio after intraluminal injection of p31-43 in C3H-HeJ
mice (Fig. 2) excluding a role for TLR4 signalling pathway.
Inflammation by p31-43 is type I interferone- (IFN) dependent
Type I IFNs may be implicated in the pathogenesis of CD [1]. To evaluate whether
type I IFNs are involved in the mechanism of damage in our model, type I IFN
receptor (IFNαR) KO mice were intraluminally injected with p31-43 and
morphometric parameters assessed in intestinal sections. No changes in V/C ratio or
IELs were observed in IFNαR KO mice treated with p31-43 compared with PBStreated
mice (Fig. 3) suggesting that type I IFN signalling pathway may play a key role
in the inflammatory changes induced by p31-43.
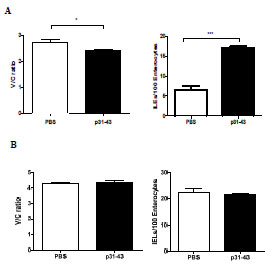
Figure 1. Mucosal changes induced by p31-43 are MyD88-dependent.
Evaluation of V/C ratio and number of IELs in small intestine sections obtained from
C57BL/6 wild type mice (A) and MyD88KO mice (B), 16 h post intraluminal injection
of PBS or p31-43. N = 5 mice per group, ANOVA and unpaired t-test *p<0.05,
***p<0.001 were performed
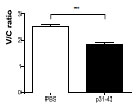
Figure 2. Mucosal changes induced by p31-43 are TLR4-independent.
Evaluation of V/C ratio in small intestine sections obtained from C3H-HeJ mice 12 h
after intraluminal administration of PBS or p31-43. N = 4 mice per group, unpaired ttest
***p≤0.001
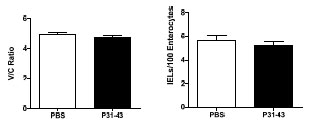
Figure 3. Mucosal changes induced by p31-43 are type I IFN dependent.
Evaluation of V/C ratio and IELs number in small intestine sections obtained from
IFNαR KO mice, 16 h post intraluminal injection of PBS or p31-43, n = 5 mice per
group. ANOVA was performed
Conclusions
Our results confirm the previous observation that intraluminally delivered p31-43
causes inflammation in an experimental murine model and expands those observations
showing that MyD88 and type I IFN signalling are required for the in vivo effects
observed. Though a receptor for the p31-43 has not been identified yet, our results
indicate that MyD88 and type I IFN participate in innate immune activation in vivo
leading to inflammation and epithelial damage. It is possible that activation of these
signalling cascades contributes to intestinal inflammation, tissue remodelling and cell
death which could impact on the development of enteropathy in a genetically
susceptible host.
References
1. Sollid LM, Jabri B. Triggers and drivers of autoimmunity: lessons from coeliac
disease. Nat Rev Immunol 2013; 13: 294-302.
2. Molberg O, Mcadam SN, Körner R, et al. Tissue transglutaminase selectively
modifies gliadin peptides that are recognized by gut-derived T cells in celiac
disease. Nat Med 1998; 4: 713-7.
3. Barone M, Troncone R, Auricchio S. Gliadin peptides as triggers of the
proliferative and stress/innate immune response of the celiac small intestinal
mucosa. Int J Mol Sci 2014; 15: 20518-20537.
4. Maiuri L, Ciacci C, Ricciardelli I, et al. Association between innate response to
gliadin and activation of pathogenic T cells in coeliac disease. Lancet 2003; 362:
30-7.
5. Hüe S, Mention JJ, Monteiro RC, et al. A direct role for NKG2D/MICA
interaction in villous atrophy during celiac disease. Immunity 2004; 21: 367-77.
6. Barone MV, Gimigliano A, Castoria G, et al. Growth factor-like activity of
gliadin, an alimentary protein: implications for coeliac disease. Gut 2007; 56: 480-
8.
7. Barone MV, Nanayakkara M, Paolella G, et al. Gliadin peptide P31-43 localises to
endocytic vesicles and interferes with their maturation. PLoS One 2010; 5:
e12246.
8. Korneychuk N, Meresse B, Cerf-Bensussan N. Lessons from rodent models in
celiac disease. Mucosal Immunol 2015; 8: 18-28.
9. Ju JM, Marietta EV, Murray JA. Generating Transgenic Mouse Models for
Studying Celiac Disease. Methods Mol Biol 2015; 1326: 23-33.
10. Araya R, Jury J, Verdu E, et al. Intraluminal p31-43 gliadin peptide induces
enteropathy. In: Koehler P (ed): Proceedings of the 28th Meeting, Working Group
on Prolamin Analysis and Toxicity. Deutsche Forschungsanstalt für
Lebensmittelchemie, Freising, 2015; pp. 99-104. ISBN: 978-3-938896-92-1.
11. Araya R, Jury J, Bondar C, et al. Intraluminal administration of poly I:C causes an
enteropathy that is exacerbated by administration of oral dietary antigen. PloS One
2014; 9: e99236.
12. Thomas KE, Sapone A, Fasano A, et al. Gliadin stimulation of murine
macrophage inflammatory gene expression and intestinal permeability are
MyD88-dependent: Role of the innate immune response in celiac disease. J
Immunol 2006; 176: 2512-2521.
5.3 The prospective multicentre trial of antibody
diagnostics in paediatric coeliac disease (AbCD):
Status update after registration of 835 children
Thomas Mothes1, Johannes Wolf1, David Petroff2, Dirk Hasenclever3
1 Institute for Laboratory Medicine, Clinical Chemistry & Molecular Diagnostics,
Medical Faculty of the University and University Hospital, Leipzig, Germany
2 Centre for Clinical Trials, University of Leipzig, Germany
3 Institute for Medical Informatics, Statistics and Epidemiology, University of Leipzig,
Germany
Introduction
Already in 2012, the ESPGHAN proposed a biopsy-sparing guideline [1] for diagnosis
of coeliac disease (CD) and stressed that the performance of these guidelines in
clinical practice should be evaluated prospectively. Since then, a number of papers
have been published on this topic, however, all of them describe retrospective studies
[2-8]. Retrospective studies have serious limitations. Most of them were performed in
coeliac-enriched populations with a high prevalence of CD and controls are sometimes
missing completely. The patients included in these studies were preselected by
previous antibody assays. In most cases, only the classical IgA antibodies against
tissue transglutaminase (IgA-anti-TTG), were evaluated, but the performance of IgG
antibodies against deamidated gliadin peptides (IgG-anti-DGP) were not considered.
In October 2012, the prospective multicentre trial of antibody diagnostics in paediatric
coeliac disease (AbCD) was launched [9]. The primary objective of this trial is to
validate a previously published [8] diagnostic cascade in patients with clinical
suspicion of CD in which many biopsies can be avoided if antibody results are
sufficiently clear. The basic trial flow and a blinded status report were already
presented one year ago [10]. Here, after registration of 835 children, an update is
given.
This is an ongoing trial and antibody results, clinical symptoms, duodenal histology
and diagnosis can only be correlated after the last patient has finished the trial. Thus,
after description of the current status of patient registration we will confine our report
to the comparison of antibody test results from test kits of different manufacturers and
on the course of antibody concentrations during follow-up.
Current status
From the 835 patients registered (key date September 14, 2015), an endoscopy was
already documented from 778 children. Of them, 630 children have already been
diagnosed. Currently, there are 393 CD patients, 228 controls, and 9 “unclear”children, in whom a decision “CD: yes or no?” could not be made. The prevalence of
CD was 62.4%.
Comparison of antibody test results from test kits of different manufacturers
The problem of differences between the antibody test kits was already addressed by
the ESPGHAN [1]. The authors concluded in the appendix of their paper that “these
results are regarded as an example for the characteristics of different tests and final
conclusions could be drawn only from more systematic studies or from a longer
survey”.
In our trial, 7 different test kits for measurement of IgA-anti-TTG were applied in the
local trial centres at the time of endoscopy and after follow-up. We compared the
results obtained by the five most commonly used local tests with the results of the test
of EUROIMMUN performed centrally (Tab. 1). We asked in how many cases the
centrally performed trial test was above and the local test below the company cut-off
and vice versa. The second question was in how many cases the centrally performed
trial test was above and the local test below a higher cut-off. As higher cut-off, 10
times above the upper limit of normal (10 x ULN) was used.
Table 1. Comparison of results of different test kits measuring IgA-antibodies towards
tissue transglutaminase.
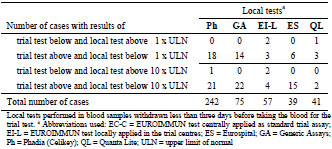
Our data confirm the view that comparison of results obtained by different test kits is
problematic [11]. Therefore, each test kit has to be calibrated individually for its
performance at different cut-offs.
Course of antibody concentrations during follow-up
There were 362 children with complete data sets for IgA-anti-TTG and IgG-anti-DGP
after follow-up (key date September 14, 2015). Of these, 334, 266, or 257 children had
initial concentrations of IgA-anti-TTG, IgG-anti-DGP, or of both antibodies,
respectively, above the cut-off. During follow-up (17 to 507 days, median 109 days),the antibody concentration decreased below the cut-off in 35.0% of the children for
IgG-anti-DGP, but in only 15.3% for IgA-anti-TTG (Tab. 2).
Alternatively one can calculate how often the concentration was reduced by more than
half of the initial value. The proportion of these patients was much larger (87.7% for
IgA-anti-TTG and 70.0% for IgG-anti-DGP).
Table 2. Decrease in antibody concentration during follow-up.
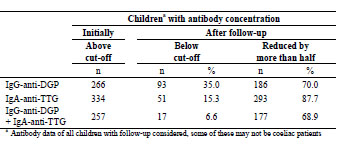
Conclusions
The final aim to include more than 900 children in the trial by the end of 2015 is
achievable. Antibody data will be correlated with clinical symptoms, duodenal
histology, and diagnoses only after the last patient has passed through the trial.
Therefore, no results on the diagnostic performance of antibody assays can be
presented now. Nevertheless, our data confirm the view that comparison of results of
antibody assays obtained by different test kits is problematic. Special efforts for
standardization of antibody assays are necessary. Thus, the data expected in the trial
can only be valid for the trial test kits.
For detection of serological remission, the ESPGHAN suggests waiting “until the
antibody titres fall below the cutoff” [1]. Our follow-up results show that it may be
better to consider the relative instead of absolute antibody concentrations. We should
keep in mind that earlier detection of serological remission spares additional later
blood withdrawals! The concentration ratio indicates remission earlier than waiting for “negativity”. For calculation of a concentration ratio, however, identical tests for initial
and follow-up measurement have to be used. Additionally, high antibody
concentrations have to be measured exactly and not expressed as concentrations above
measuring range (or out of range). If no initial values with the same antibody test are
available, IgG-anti-DGP may be a better indicator for remission than IgA-anti-TTG.
Acknowledgements
Thanks to the clinical investigators Th. Richter (Children’s Hospital of the Clinical
Centre “Sankt Georg”, Leipzig, Germany), H. Uhlig (Translational Gastroenterology
Unit, Experimental Medicine, University of Oxford, John Radcliffe Hospital, Oxford,
England), M. Auth (Alder Hey Children’s National Health Service Foundation,
Liverpool, England), M. Laaß (University Children’s Hospital, Dresden, Germany),
K.-M. Keller (Department of Paediatrics, Deutsche Klinik für Diagnostik, Wiesbaden,
Germany), N. Händel (University Children’s Hospital, Leipzig, Germany), A. Krahl
(Children’s Hospital “Prinzessin Margaret”, Darmstadt, Germany), A. Hauer
(University Children’s Hospital, Graz, Austria), K.-P. Zimmer (University Children’s
Hospital, Gießen, Germany), G. Flemming (Children’s Hospital of Hannover Medical
School), F. Schmidt (University Children’s Hospital, Halle, Germany), M. Heiduk
(Department of Paediatrics, Helios Hospital, Plauen, Germany), W.-D. Huber
(University Children’s Hospital, Vienna, Austria), to the reference pathologists V.
Wiechmann (Department of Pathology of the Clinical Centre “Sankt Georg”, Leipzig,
Germany), D. Aust (Department of Pathology, University Hospital, Dresden,
Germany), A.-K. Höhn (Department of Pathology, University Hospital, Leipzig,
Germany), and to B. Teegen and A. Jahnke (EUROIMMUN Medizinische
Labordiagnostika AG, Niederlassung Dassow) for antibody assays. This trial is part of
a project funded by the European Regional Development Fund.
References
1. Husby S, Koletzko S, Korponay-Szabo IR, et al. European Society for Pediatric
Gastroenterology, Hepatology, and Nutrition Guidelines for the Diagnosis of
Coeliac Disease. J Ped Gastroenterol Nutr 2012; 54: 136-160.
2. Beltran L, Koenig M, Egner W, et al. High-titre circulating tissue
transglutaminase-2 antibodies predict small bowel villous atrophy, but decision
cut-off limits must be locally validated. Clin Exp Immunol 2013; 176: 190-198.
3. Donat E, Ramos JM, Sánchez-Valverde F, et al. ESPGHAN 2012 guidelines for
coeliac disease diagnosis: Validation through a retrospective Spanish multicentric
study. J Pediatr Gastroenterol Nutr 2016; 62: 284-291.
4. Gidrewicz D, Potter K, Trevenen CL, et al. Evaluation of the ESPGHAN Celiac
Guidelines in a North American Pediatric Population. Am J Gastroenterol 2015;
110: 760-767.
5. Oyaert M, Vermeersch P, de Hertogh G, et al. Combining antibody tests and
taking into account antibody levels improves serologic diagnosis of celiac disease.
Clin Chem Lab Med 2015; 53: 1537-1546.
6. Suh-Lailam BB, Davis KW, Tebo AE. Immunoassays for the detection of IgA
antibodies to tissue transglutaminase: Significance of multiples of the upper limit
of normal and inter-assay correlations. Clin Chem Lab Med 2016; 54: 257-264.
7. Trovato CM, et al. Are ESPGHAN “Biopsy-Sparing” Guidelines for Celiac
Disease also Suitable for Asymptomatic Patients? Am J Gastroenterol 2015; 110:
1485-1489.
8. AbCD – Clinical Trial. Available: https://eclinical.imise.uni-leipzig.de/abcd/
9. Wolf J, Hasenclever D, Petroff D, et al. Antibodies in the diagnosis of coeliac
disease: A biopsy-controlled, international, multicentre study of 376 children with
coeliac disease and 695 controls. PLoS ONE 2014; 9: e97853.
10. Mothes T, Wolf J, Petroff D, et al. The prospective multicentre trial of antibody
diagnostics in paediatric coeliac disease (AbCD) - blinded status report after
registration of 437 children. In: Koehler P (ed): Proceedings of the 28th meeting,
Working Group on Prolamin Analysis and Toxicity. Deutsche Forschungsanstalt
für Lebensmittelchemie, Freising, 2015; pp. 87-91
11. Egner W, Shrimpton A, Sargur R, et al. ESPGHAN Guidance on Coeliac Disease
2012: Multiples of ULN for Decision Making Do Not Harmonise Assay
Performance Across Centres. J Pediatr Gastroenterol Nutr 2012; 55: 733-735.
5.4 Liver tissue as an alternative substrate for
endomysium antibody testing in diagnosis of
paediatric coeliac disease
Johannes Wolf1, Annika Jahnke2, Thomas Richter3, Holm H Uhlig4, Martin W. Laass5,
Almuthe Hauer6, Martin Stern7, Jan de Laffolie8, Gunter Flemming9, Thomas Mothes1
1 Institute of Laboratory Medicine at the University Hospital, Leipzig, Germany
2 EUROIMMUN Labormedizinische Diagnostika AG, Lübeck, Germany
3 Children’s Hospital of the Clinical Centre “Sankt Georg”, Leipzig, Germany
4 John Radcliffe Hospital, Oxford, United Kingdom
5 University Children’s Hospital, Dresden, Germany
6 University Children’s Hospital, Graz, Austria
7 University Children’s Hospital, Tübingen, Germany
8 University Children’s Hospital, Gießen, Germany
9 University Children’s Hospital, Leipzig, Germany
Introduction
Immunofluorescence assays of antibodies against endomysium (EmA) still represent
the gold standard in the serological testing for coeliac disease (CD). EmA are
traditionally detected on oesophagus sections of primates. EmA bind to the connective
tissue layers around the smooth muscle fibres of the Lamina muscularis mucosae and
Tunica muscularis of oesophagus sections [1]. Other tissues, for instance human
umbilical cord, were proposed as alternative substrate but became not accepted [2].
Nevertheless, EmA were gradually replaced in the last decade by ELISA tests
detecting IgA and/or IgG antibodies against tissue transglutaminase (IgA-tTG or IgGtTG),
which is the main autoantigen in the connective tissue of oesophagus [3].
Currently, IgA-EmA are enjoying a diagnostic renaissance due to the recommendation
as a confirmatory test in the ESPGHAN guidelines for diagnosis of CD [4].
The widespread tissue transglutaminase is also abundant in the endothelia of nearly
each tissue [5]. Therefore, liver seems to be good substrate due to the high number of
blood vessels/capillaries, called sinusoids. It is notable that some laboratories already
use liver instead of oesophagus for EmA detection. But there is currently no study,
which examined systematically the application of liver for EmA detection. Therefore,
we analysed a retrospective dataset, in which liver tissue was used in parallel to
oesophagus as substrate for EmA.
Materials and methods
We analysed serum from 872 children (298 CD patients and 574 disease controls, 379
boys and 493 girls, mean age 8.9 years, range 0.3 to17.9 years). The patients were
recruited consecutively from six centres in Germany and Austria between 2004 and
2013. All patients were biopsied under gluten-containing diet due to suspicion of CD
or other gastrointestinal disorders. The diagnosis of CD was considered confirmed in
patients with Marsh 2 lesions and higher. Serum was collected around the time of
duodenal biopsy under gluten-containing diet and was stored until measurement at
-20°C. The study was approved by the ethical committee of the University of Leipzig
and of the local ethical committees of the participating centres.
Sera for detection of IgA-EmA and IgG-EmA were diluted as described by the
manufacturer (1:10 to 1:10,000) and incubated in parallel on commercial slides with
acetone-fixed cryostat sections of monkey oesophagus and liver tissue from
EUROIMMUN Medizinische Labordiagnostika AG (Lübeck, Germany). The cut-off
was ≥1:10, respectively. All further incubation and wash-steps were performed as
described in the test manual. Immunofluorescence patterns were estimated by using an
Eurostar Plus microscope with Bluelight LED (EUROIMMUN Medizinische
Labordiagnostika AG, Lübeck, Germany). Assessment of EmA titre on oesophagus
and liver was performed consecutively by experienced observers and blinded to
diagnosis and histology. IgG-tTG was also tested in all sera as described by the manufacturer
(EUROIMMUN Medizinische Labordiagnostika AG, Lübeck, Germany).
Table 1. Performance of IgA-EmA on oesophagus and liver tissue sections.
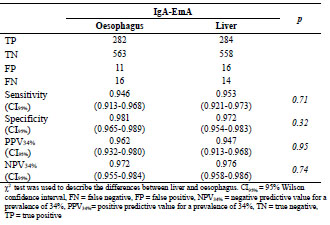
We used maximum likelihood estimates for sensitivity, specificity, positive (PPV) and
negative predictive value (NPV). The 95% Wilson confidence intervals for sensitivity,
specificity, PPV and NPV were determined based on a normal approximation to avoid
being overly optimistic [6]. Calculations and graphics were produced with SPSS 20. χ2
test was used to describe the differences between liver and oesophagus.
Results and discussion
The results of IgA- and IgG-EmA testing on liver in comparison with oesophagus are
depicted in Tab. 1 and 2, respectively.
For IgA-EmA (Tab. 1), oesophagus and liver sections showed comparable sensitivities
(0.946 vs 0.953) and specificities (0.981 vs 0.972). The predictive values (PPV and
NPV) calculated for the prevalence of CD in our study cohort (34%) exhibited also no
significant difference estimating IgA-EmA (0.962 vs 0.947 and 0.972 vs 0.976,
respectively). These data show that liver is a substrate as good as oesophagus for assay
of IgA-EmA.
Quite contrary to this, an additional number of 23 CD patients were positive for IgGEmA
on liver tissue (Tab. 2). This results in a significantly increased sensitivity (0.517
vs 0.629; p = 0.006).
Table 2. Performance of IgG-EmA on oesophagus and liver tissue sections and of
ELISA for IgG-tTG.
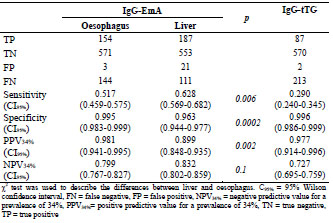
Simultaneously, we observed a significant decrease in the specificity (0.995 vs 0.963;
p = 0.002) and PPV (0.981 vs 0.899; p = 0.0002) on liver compared to oesophagus
caused by a 7-fold higher rate of control patients with positive IgG-EmA test on liver
tissue. Despite the significant increase in sensitivity, liver tissue should not be applied
for IgG-EmA detection due to the noticeable decrease of PPV.
The low sensitivity of IgG-EmA assay on both tissues of 52 to 63%, however, is still
superior to IgG-tTG assay by means of ELISA (29%). These data do not support the
strategy of most laboratories to assay IgG-tTG instead of IgG-EmA.
Autoantibody binding patterns can be misinterpreted due to occurrence of other “unspecific” autoantibodies which overlap with the CD typical staining patterns. This
was also observed during assessment of possible EmA staining. Typical staining
patterns of EmA both on liver and oesophagus are illustrated in Fig. 1A and D.
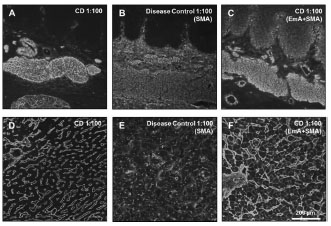
Figure 1. Staining patterns of IgA autoantibodies on liver and oesophagus sections.
Primate oesophagus (A-C) and primate liver (D-F) sections after incubation with
serum of a coeliac disease (CD) patient with high autoantibody concentrations (A and
D), of a patient with only anti-smooth muscle antibodies (SMA, B and E), and of a CD
patient with both, IgA-EmA and SMA (C and F)
Using oesophagus, other autoantibodies such as anti-smooth muscle antibodies (SMA)
bind to structures similar to those recognized by EmA (Fig. 1B). This mimics EmA
and can produce a false positive result. The staining pattern of SMA on liver tissue was clearly different from that of EmA (Fig. 1E). In detail, SMA stained intracellular
filaments whereas EmA bound to endothelia of sinusoids. If both antibodies co-exist in
one serum sample, and in case of high SMA concentration, these antibodies may hide
EmA staining on oesophagus (Fig. 1C). On liver tissue, both antibody species can be
clearly distinguished (Fig. 1F).
Conclusions
The abundance of tissue transglutaminase in endothelia cells has been known since a
long time. Therefore, the use of liver, which is rich in capillaries, might be a good
substrate for autoantibodies against tissue transglutaminase. We show that the
performance of the IgA-EmA test on liver and oesophagus is comparable, whereas the
assay of IgG-EmA on liver has higher sensitivity, but slightly lower specificity as well
as a noticeably lower PPV than on oesophagus. Therefore, IgG-EmA on oesophagus
should still be favoured. The IgG-tTG ELISA seems to be unsuitable due to its low
sensitivity. Instead, IgG-EmA on oesophagus is recommended.
Further, an advantage of the liver sections may be that interpretation of the staining is
easier than on oesophagus sections, since there is less interference on liver by other
autoantibodies (e.g. SMA). To sum up, our study shows that liver is an alternative
substrate to oesophagus in detection of IgA-EmA.
References
1. Chorzelski TP, Beutner EH, Sulej J, et al. IgA anti-endomysium antibody. A new
immunological marker of dermatitis herpetiformis and coeliac disease. Br J
Dermatol 1984; 111: 395-402.
2. Castellino, F, Scaglione N, Grosso SB, et al. A novel method for detecting IgA
endomysial antibodies by using human umbilical vein endothelial cells. Eur J
Gastroenterol Hepatol 2000; 12: 45-49.
3. Dieterich, W, Laag, E, Schopper H, et al. Autoantibodies to tissue
transglutaminase as predictors of celiac disease. Gastroenterology 1998; 115:
1317-1321.
4. Husby S, Koletzko S, Korponay-Szabó I, et al. European Society for Pediatric
Gastroenterology, Hepatology, and Nutrition Guidelines for the Diagnosis of
Coeliac Disease. J Pediatr Gastroenterol Nutr 2012; 54:136-60.
5. Lorand L, Graham RM. Transglutaminases: crosslinking enzymes with pleiotropic
functions. Nat Rev Mol Cell Biol 2003; 4:140-156.
5.5 Mass cytometry: high-dimensional dissection of the
immune system reveals previously unrecognized
heterogeneity
Frits Koning
Department of Immunohaematology and Blood Transfusion, Leiden University
Medical Centre, Leiden, The Netherlands
Our immune system protects us from infections by invading pathogens such as
bacteria, viruses and helminths [1]. To achieve this task our immune system is not only
capable of producing antibodies that will help to neutralize and/or eliminate pathogens
from the circulation, it is also capable of recognizing and destroying virus-infected
cells. In addition, our immune system develops memory: upon a first encounter with a
particular pathogen, the immune system evolves such that upon re-exposure to the
same or a highly related pathogen a more rapid response can be mounted, often
eliminating the invader before any harm can be done. However, the immune system
can also mount unwanted responses directed to self-antigens, resulting in a variety of
immune-mediated diseases like type 1 diabetes and rheumatoid arthritis. Also,
exaggerated immune response to the microbiota in the intestine can lead to chronic
inflammatory bowel diseases like Crohn’s disease, often with grave consequences.
Finally, inadvertent immune response to food antigens can result in a variety of
diseases, including coeliac disease, caused by immune responses to the gluten proteins
in wheat [2].
To execute its functions the immune system uses a variety of cell types, each with their
own specialized function, in concert with soluble factors, again each with its own welldefined
function [1]. “Classically” the immune system can be subdivided in 6 major
lineages: CD4 T cells, CD8 T cells, γδ T cells, B cells, myeloid cells and innate
lymphocytes (including NK cells) [1]. The innate lymphocytes form a first line of
defence. CD4 T cells are known as helper T cells, they orchestrate the immune
responses. CD8 T cells are efficient in killing pathogen-infected cells. B cells produce
antibodies, soluble factors that help to eliminate pathogens from the circulation and
myeloid cells can internalize and kill pathogens. γδ T cells exert a variety of functions,
not all properly understood. Every cell subset is distinguished by the expression of
particular cell surface molecules like CD4 in the case of CD4 T cells and CD8 in case
of CD8 T cells. Thus, generally CD4 T cells will express CD4 but not CD8 and vice
versa. Over 250 CD markers have now been identified. In addition, a large number of
intracellular markers are known, including highly lineage-specific transcription
factors. Immunohistochemistry and flow cytometry are the two major techniques that
are employed to determine the expression of CD and intracellular antigens by immune
subsets of interest. The power of immunohistochemistry is that cells can be studied in
their natural environment: in tissue sections. The downside is that only a few marker proteins can be visualized simultaneously. Flow cytometry allows the simultaneous
visualization of up to 15 markers on single cells and therefore has become a much
used technique in both diagnostics and research. Through flow cytometric analysis of
the immune system it has become evident that its composition is much more complex
than described above. All 6 major lineages can be subdivided into multiple
sublineages, each with a distinct marker expression profile. For example, several
distinct CD4 subsets can readily be identified through the expression of a membrane
protein that distinguished naïve CD4 T cells from memory CD4 T cells. Similarly,
pro-inflammatory and regulatory CD4 T cell subsets can be defined. More recently,
the innate lymphocyte compartment was found to harbour a variety of cellular subsets
with regulatory properties as well [3,4].
However, flow cytometry has its limitations as the number of antigens that can be
monitored simultaneously is limited due to the spectral overlap between fluorochromes
used to label the antibodies used. This limitation has recently been overcome due to
the introduction of mass cytometry in which flow cytometry is combined with mass
spectrometry. Instead of labelling the antibodies with fluorochromes, rare earth metals
are used [5,6], of which some 100 are available, all of which can be distinguished from
each other by mass spectrometry. In principle, therefore, up to 100 antibodies can each
be uniquely labelled and combined to stain (immune) cells, followed by data
acquisition on the CyTOF, the mass cytometer. At present up to 36 antibodies can be
routinely applied through this methodology, offering a significant advance over
conventional flow cytometry.
We have applied a 32 antibody panel to analyse the composition of the immune system
in detail. In the analysis, we compared peripheral blood samples with matched
intestinal samples from the intestine. Single cells suspensions were prepared and
stained with the antibody panel and analysed on the CyTOF. Special computational
tools and a data driven approach were applied to accommodate the high-dimensional
data sets acquired. The results reveal previously unrecognized heterogeneity within the
immune system as over 100 distinct immune subsets were identified, some of which
were tissue- or disease-associated. Currently, the antibody is being optimized and
expanded which is expected to increase the resolution of the approach even more. It is
anticipated that through this technology diagnostic procedures can be significantly
improved. Such analyses may also help to predict and/or monitor the response to
therapeutic approaches, an important step towards personalized medicine.
References
1. Parham P. The Immune System. Garland Science, New York, 2009; ISBN: 978-0-
8153-4146-8, 3rd ed.
2. Tjon JM, van Bergen J, Koning F. Celiac disease: how complicated can it get?
Immunogenetics 2010; 62: 641-51.
3. Spits H, Cupedo T. Innate lymphoid cells: emerging insights in development,
lineage relationships, and function. Annu Rev Immunol 2012; 30: 647-675.
4. Hazenberg MD, Spits H. Human innate lymphoid cells. Blood 2014; 124: 700-709.
5. Bendall SC, Simonds EF, Qiu P, et al. Single-cell mass cytometry of differential
immune and drug responses across a human hematopoietic continuum. Science
2011; 332: 687-96.
6. Becher B, Schlitzer A, Chen J, et al. High-dimensional analysis of the murine
myeloid cell system. Nat Immunol 2014; 15: 1181-1189.
5.6 Gliadin and avenin toxicity studies of duodenal biopsy
tissue in organ culture experiments
Sarah Cooper1, Jacinta Kelly2, Conleth Feighery1,3
1 Immunology Department, TCD, Dublin
2 National Children’s Research Centre, Our Lady’s Children’s Hospital, Dublin
3 Immunology Department, St James’s Hospital, Dublin
Introduction
Oats is phylogenetically more distantly related to the other food cereals. This led to the
original in vivo oats challenge studies, which reported that oats did not cause activation
of coeliac disease [1,2]. Further studies of paediatric and adult coeliac patients
supported these earlier observations. However, other investigators reported that oats
could activate coeliac disease in some patients and suggested that if oats were taken in
sufficient quantity over a longer time period, the cereal might prove to be toxic [3,4].
Because of the continuing interest in possible oats toxicity, we performed a further
year long in vivo oats challenge study in 54 patients and none showed adverse effects
to this cereal [5]. In addition, we carried out in vitro studies examining the possible
toxic effect of wheat gliadin or oats avenin (as peptic/tryptic digests) when added to
duodenal biopsy tissue in organ culture experiments. These latter experiments are
reported here.
Materials and methods
Patients
Six control patients and five treated coeliac patients were recruited for the study and
approval was given by the St. James’s Hospital Ethics Committee. All patients gave
informed consent. Duodenal biopsy tissue from each were cultured as described
below. In addition, two biopsies from each patient were placed directly into formalin
as time 0 controls.
Prolamin fractions
The prolamin fractions used in the study were kindly prepared by Prof. Peter Koehler
(Deutsche Forschungsanstalt für Lebensmittelchemie, Freising, Germany). Wheat
gliadin was a gift from the Working Group on Prolamin Analysis and Toxicity
(“PWG-gliadin”). The prolamin fraction from oats (avenin) was derived from the
German oats cultivar “Scorpion”. Peptic-tryptic (PT) digests of each prolamin were
prepared and stored as a freeze-dried product. In preparation for culture, 5 mg/mL PT
gliadin and avenin were re-suspended separately in RPMI-1640 medium supplementedwith 15% heat inactivated, filtered foetal calf serum and a 1% antibiotic/antimycotic
solution.
Organ Culture
Pairs of biopsies collected in RPMI were orientated villous side up on a nylon mesh
filter. 1.5 mL of PT avenin, PT gliadin or RPMI was added to the central well of a
culture dish and the filter with biopsies was placed in the central well so that the
biopsies were in contact with the surface of the medium. The remainder of the aliquot
of PT avenin, PT gliadin or RPMI was dotted around the outer well of the culture dish.
Culture dishes were placed in a modular incubator chamber, gassed with 95% O2 / 5%
CO2 and placed in a 37 °C incubator. Following 24 h of culture, biopsies were
transferred to histology cassettes and immersed in formalin. All 8 biopsies were
formalin-fixed and paraffin-embedded.
Immunofluorescent staining
4-m-thick tissue sections were incubated with a mouse monoclonal antibody to Ecadherin
conjugated to Alexafluor488 and a rabbit monoclonal antibody to Cytokeratin
20 at 4 °C, overnight. Sections were then incubated with an Alexafluor568-conjugated
secondary GAR antibody for 1 h at RT. Tissue sections were incubated with
phosphate-buffered saline (PBS) instead of Cytokeratin 20 and a mouse monoclonal
antibody to alpha-actin conjugated to Alexafluor488 instead of E-cadherin conjugated
to Alexafluor488, to check that staining was specific. To visualize nuclei, all sections
were incubated with Hoechst 33258. All sections were examined and imaged under a
confocal microscope at 63x magnification.
Results and discussion
General effects of biopsy culture
The culture process affected all biopsies, regardless of whether they came from a
control or a coeliac patient. Immediately after biopsy, the tissue had a plump and
rounded appearance and biopsies placed directly into formalin retained that
configuration. However, after 24 h culture on a mesh filter, the other biopsies were
visibly flattened and generally difficult to separate from the mesh filter. In these
biopsies the villi were generally shorter and wider and often folded over on
themselves. Enterocytes were also observed to be much shorter in cultured biopsies
compared to time 0 biopsies.
Control patients
All time 0 biopsies from control patients displayed healthy, elongated enterocytes (Fig.
1). Orderly patterns of E-cadherin staining were present with narrow, generally
straight lines of green fluorescence visible between enterocytes. In some biopsies
cytokeratin 20 staining was visible throughout the enterocytes; however, in others the quality of staining was suboptimal (the cytokeratin staining is not visible in the
greyscale photographs used in this publication). Following 24 h in culture, although
enterocytes were considerably shorter, no patient showed any signs of a reaction to
culture with PT gliadin or PT avenin. The appearance of enterocytes and nuclei
remained organised and the pattern of E-cadherin expression remained similar to that
observed at time 0. Although, cytokeratin 20 staining was of good quality only in some
biopsies, there was no evidence of an effect caused by prolamins.
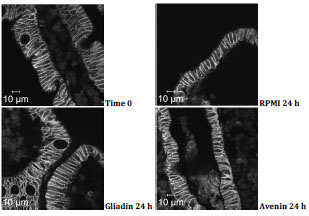
Figure 1. Control patient. E-cadherin, cytokeratin 20 and Hoechst staining at time 0
and after 24 hours in culture with RPMI, PT gliadin or PT avenin
Coeliac patients
At time 0, three of the five coeliac patients had a routine histological appearance
consistent with that of treated CD. Two further patients were reported to have
increased IELs and villous blunting. All five coeliac patients had negative tTG
serology. Following culture with RPMI, strong E-cadherin staining was still visible
between enterocytes. Again, cytokeratin 20 staining was of poor quality in all five
subjects. After 24 h culture in the presence of PT gliadin four out of five patients
showed signs of damage (Fig. 2, arrows). In addition to shortening of enterocytes, Ecadherin
staining was less consistent. Although in some areas, biopsies still displayed
healthy looking enterocytes, in other areas the pattern of E-cadherin expression was
altered, with loss of the nice, straight line of green E-cadherin staining between
enterocytes.
The addition of PT avenin did not appear to have any significant effect on biopsies
from two of the five coeliac patients (Fig. 2). Similarly to culture with RPMI,
enterocytes were shortened but remained generally healthy looking and had normal
expression of E-cadherin. In contrast, biopsies from three of the coeliac patients
showed evidence of change; although there were some healthy looking enterocytes,
there were also areas where enterocytes were less well organised and they lacked the
consistent expression of E-cadherin (Fig. 3, arrows). This appearance was similar to
that observed with PT gliadin.
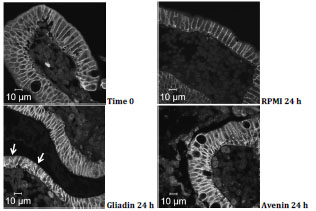
Figure 2. Coeliac patient. E-cadherin, cytokeratin 20 and Hoechst staining at time 0
and after 24 hours in culture with RPMI, PT gliadin or PT avenin. Arrows indicate
shortening of enterocytes and patchy E-cadherin staining
In this study, we attempted to develop an in vitro assay which would examine the
question of whether a prolamin fraction from oats caused damage to coeliac biopsy
tissue in culture. Since enterocyte damage is an early feature of gliadin mediated
damage, we employed E-caderin and cytokeratin 20 staining of enterocytes with
confocal microscopy to investigate this question. In the case of tissue from normal
controls, there appeared to be no evidence of damage caused by the prolamin fractions.
Gliadin caused changes in E-cadherin staining in four of the five coeliac subjects.
However, avenin appeared to cause similar changes in three of the five coeliac biopsy
experiments. Unfortunately, cytokeratin 20 staining was too unreliable to add
meaningful information to the studies.
Conclusions
The experimental technique described here may be useful in determining the potential
toxicity (as opposed to immunogenicity) of prolamin fractions or cereal peptide
sequences. However, further optimisation of the technique is required and there is a
need to overcome the inherent subjectivity, involved in the interpretation of tissue
changes.
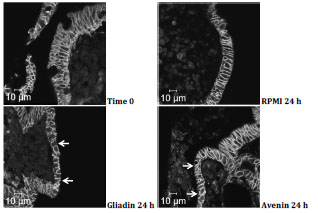
Figure 3. Coeliac patient. E-cadherin, cytokeratin 20 and Hoechst staining at time 0
and after 24 hours in culture with RPMI, PT gliadin or PT avenin. Arrows indicate
disorganization and shortening of enterocytes and patchy E-cadherin staining
References
1. Janatuinen EK, Pikkarainen PH, Kemppainen TA, et al. A comparison of diets
with and without oats in adults with celiac disease. N Engl J Med 1995; 333 (16):
1033-7.
2. Srinivasan U, Leonard N, Jones E, et al. Absence of oats toxicity in adult coeliac
disease. BMJ 1996; 313: 1300-1.
3. Lundin KEA, Nilsen EM, Scott HG, et al. Oats induced villous atrophy in coeliac
disease. Gut 2003; 52: 1649-52.
4. Arentz-Hansen H, Fleckenstein B, Molberg Ø, et al. The molecular basis for oat
intolerance in patients with celiac disease. PLoS Med 2004; 1: e1.
5. Cooper SEJ, Kennedy NP, Mohamed BM, et al. Immunological indicators of
coeliac disease activity are not altered by long-term oats challenge: oats challenge
does not alter immunological parameters in coeliac disease. Clin Exp Immunol
2013; 171: 313-8.
6 Antigen receptors in coeliac disease
6.1 Gliadin peptides as trigger of the stress/innate
immune response of the coeliac small intestine
Merlin Nanayakkara, Giuliana Lania, Riccardo Troncone, M. Vittoria Barone
Department of Translational Medical Science and European Laboratory for the
Investigation of Food-Induced Diseases, University Federico II, Naples, Italy
Introduction
Ingested food can cause tissue inflammation through different mechanisms. In the
intestine, and particularly in the enterocyte, nutrients are modulators of various cellular
functions and may be involved in tissue immune response and inflammation [1]. An
example of an intestinal inflammatory and remodelling response of the intestine to
food is the small intestinal coeliac lesion induced by gluten, an alimentary protein
present in wheat and other cereals. Coeliac disease (CD) is characterized by
inflammatory and structural changes resulting in remodelling of the small intestinal
mucosa [2].
Gliadin, the major storage protein component of wheat, is a peculiar protein very rich
in glutamine and proline. Several gliadin peptides are recognized by T cells (TC) of
the coeliac intestine, and can induce the adaptive immune response, but most of them
are digested by gastric, pancreatic and intestinal peptidases. Several gliadin peptides
remain undigested [3]: among these the 33-mer (P55-87) and the 25-mer (P31-55).
The inflammation of the intestinal mucosa is due not only to the adaptive, but also to
the innate immune responses to wheat gliadin. The P31-43 peptide, which is contained
in the 25-mer, is not recognized by TC in the coeliac intestine and is able to damage
the coeliac intestinal mucosa in vitro and in vivo [3,4]. Moreover, the P31-43 gliadin
peptide is able to initiate both a stress and an innate immune response with interleukin-
15 (IL-15) as a major mediator [3].
P31-43 is also known to exert a number of biological activities. Although the structural
changes of the coeliac mucosa are considered a consequence of sustained mucosal
inflammation due to the Th1-TC response, recent data have shown that gliadin
peptides, in particular P31-43, induce proliferation of coeliac enterocytes. This process
is epithelial growth factor- (EGF) and IL-15-dependent, and has profound effects in
inducing the crypt hyperplasia, which is characteristic of the remodelling of the coeliac
mucosa [5-8]. Moreover, gliadin peptides induce alterations of structure (cell shape,
actin modifications, increased permeability, vesicular trafficking alterations, signalling
and proliferation and stress/innate immunity activation in several cell lines [3].
In this paper we will discuss the P31-43 activities that are linked to the activation of
the stress/innate immunity activation in the intestinal epithelial cell line (CaCo2) and
in intestinal biopsies from CD patients.
Role of P31-43 in enterocyte proliferation, structural changes and
innate immune response
Alterations of the intestinal mucosa in CD are mediated both by inflammation due to
the adaptive and innate immune response to gliadin and by proliferation of crypt
enterocytes causing crypt hyperplasia [3]. The coeliac intestine is characterized by an
inversion of the differentiation/proliferation program of the tissue, with a reduction in
the differentiated compartment, up to complete villous atrophy, and an increase in the
proliferative compartment with crypt hyperplasia [3].
Gliadin peptides and P31-43 induce cell proliferation and actin rearrangements [8,5] in
several cell lines. We have already reported [3] that in CaCo2 cells, mimicking the
effect of gliadin peptides can induce actin remodelling, proliferation and activation of
the EGF-receptor (EGFR)/extracellular signal-regulated kinases (ERK) pathway [5]
(Fig. 1). P31-43 can also induce increase of IL-15/IL-15R alpha in CaCo2 cells [3].
Interestingly, levels of IL-15 do not change after P31-43 treatment when analysed by
quantitative polymerase chain reaction (PCR) [3], but the levels of the protein present
on the cell surface increase rapidly after P31-43 treatment, as measured by
fluorescence-activated cell sorting (FACS) and western blot analysis (Fig. 1). The
increased IL-15 on the cell membrane worked as a growth factor for TLL2 cells, a cell
line that depends on IL-15 for its growth [3]. Moreover, IL-15 can induce epithelial
proliferation both in intestinal biopsies and in CaCo2 cells [8]. Interestingly, P31-43
was able to induce activation, again in CaCo2 cells, of tissue transglutaminase (tTG)
and of glucose-regulated protein 78 (GRP78), both markers of cellular stress [9].
Finally, intestinal biopsies from CD patients in the active phase of the disease, but not
from controls, increased proliferation after P31-43 treatment (Fig. 1).
Taken together all these data indicate that gliadin peptide P31-43 is able to induce
proliferation, increase of stress markers and of innate immunity activation in an
intestinal epithelial cell line and in intestinal biopsies from CD patients [3,10].
Mechanisms of IL-15 and EGF upregulation in CD induced by P31-
43: role of vesicular trafficking
The mechanisms through which P31-43 might induce the coeliac intestinal innate
immune response and EGF- and IL-15-mediated enterocyte proliferation have recently
been investigated. Recent evidence points to an effect of P31-43 on the endocytic
compartment [3].
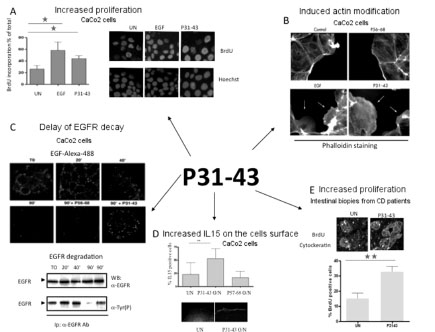
Figure 1. P31-43 effects on CaCo2 cells and intestinal biopsies.
A) P31-43 increased proliferation in CaCo2 cells. Increase of Bromodeoxiuridine incorporation
(BrdU) after treatment with P31-43 and EGF, used as positive control, respect to the untreated (UN)
sample. Statistical analysis of five independent experiments and immunofluorescence images of
CaCo2 nuclei that incorporate BrdU (red) respect to total nuclei (blue)
B) P31-43 induced actin modifications in CaCo2 cells. Immunofluorescence images of CaCo2 cells
stained with phalloidin to highlight F actin
C) Pulse-chase determination of EGFR activity. Endocytic vesicles containing EGF-Alexa488 persist
longer after P31-43 treatment of CaCo2 cells. Cells were pulsed for 1 h at 0°C with EGF-Alexa488
and chased after temperature shift to 37°C, with P31-43 or control P56-68 for the times indicated. 63x
objective. Representative results from 3 independent experiments. Western blot of immunoprecipitated
EGFR from CaCo2 cells which were pulsed for 1 h with EGF at 0°C and chased for the indicated
times after temperature shift to 37 °C. In PTG and P31-43 treated samples, the EGFR is still activated
90’ after temperature shift, by which time all activated EGFR has been degraded in control cells.
Densitometric analysis of Western blot experiments. EGFR phosphorylation is expressed as foldincrease
over the corresponding untreated line. UN=untreated
D) Gliadin peptide P31-43 increased IL-15/IL-15R alpha complex on the cell surface in CaCo-2 cells.
P31-43 increased IL-15 on the cell surface in CaCo-2 cells. FACS analysis of IL-15 on the cell surface
after overnight (O/N) P31-43 or control gliadin peptide P57-68. Statistical analysis of ten independent
experiments. Confocal images of IL-15 R-alpha expression on CaCo2 cell surfaces. 63x objective
E) P31-43 increased proliferation in intestinal biopsies from CD patients. Statistical analysis of three
independent experiments. Columns represent means and bars are the standard deviations. *= p<0.05
(Student’s t-test), **= p<0.01 (Student’s t-test).
Endocytosis has many effects on signalling; in fact, signalling pathways and endocytic
pathways are regulated in a reciprocal manner. It is now widely accepted that the "endocytic matrix" is a master organizer of signalling, governing the resolution of
signalling in space and time. Consequently, endocytosis affects several cell functions,
ranging from proliferation to actin organization, cell motility and stress/innate
immunity activation [3].
P31-43 is strikingly similar to a region of hepatocyte growth factor regulated substrate
kinase (HRS), a key molecule regulating endocytic maturation, which is localized on
the membranes of early endocytic vesicles [11]. The sequence similarity between
gliadin peptide P31-43 and HRS is in a small area of the proline/glutamine-rich
domain of HRS. The COOH terminal of HRS contains a clathrin-binding domain that
binds clathrin to clathrin-coated vesicles and is one of the domains needed to localize
HRS to the vesicle membranes. Both in CaCo2 cells and in the coeliac enterocytes,
P31-43 localizes in the early endosome and delays vesicular trafficking [3].
In CaCo2 cells, P31-43 interferes with the correct localization of HRS at the level of
the early endosomes, interfering with HRS-mediated maturation of early endosomes.
By interfering with the localization to the endocytic membrane of HRS, P31-43
induces two important effects: a) it delays endocytic maturation and b) it alters the
recycling pathway. By delaying the maturation of endocytic vesicles, P31-43 reduces
the degradation of EGFR and other RTKs (receptor tyrosine kinases) that are
endocytosed in these vesicles, and prolongs their activation, resulting in increased
proliferation, actin remodelling and other biological effects. The alteration of the
recycling pathway is able to direct more transferrin receptor and IL-15R alpha to the
cell surface, allowing more IL-15/IL-15R alpha trans-presentation in epithelial cells.
Moreover, the trans-presented IL-15 is able to activate IEL in vitro in an IL-15-
dependent way, demonstrating that the effects of P31-43 on enterocytes can activate
signalling in lymphocytes [3]. Interestingly, more IL-15R alpha is expressed in CD
enterocytes and in patients on a gluten-free diet, indicating that in the CD mucosa, a
constitutive alteration of IL-15R alpha trafficking could be present (see below).
The production of IL-15 is tightly controlled at multiple levels, not only at the level of
intracellular trafficking but also of transcription and translation. P31-43 increased IL-
15 mRNA levels only after prolonged incubation, whereas the increase of the transpresented
IL-15/IL-15R alpha complex on the cell surface was an early effect [12]. By
increasing the synthesis of IL-15 and the amount of the cytokine that is trans-presented
to the neighbouring cells, P31-43 affects both enterocyte proliferation, which is
EGFR-IL-15 dependent, and the activation of innate immunity (Fig. 2) [12].
Constitutive alterations in CD cells
Recent observations suggest an effect of P31-43 on the maturation and function of
early endocytic vesicles and consequently on EGFR signalling, enterocyte
proliferation and IL-15 trans-presentation and synthesis [3]. However, the explanation for why the stress/innate immune and proliferative responses to certain gliadin
peptides (i.e., P31-43) in the CD intestine are so intense and disruptive has not been
elucidated. For this reason, it is interesting to review the recent literature regarding
constitutive alterations in CD biopsies and cells. Several reports note constitutive,
gluten-independent alterations of the CD cells. They have been studied in the
normalized intestinal biopsies of patients in the remission phase of the disease on a
gluten-free diet and in cells obtained from tissues far away from the intestine, the
primary site of inflammation.
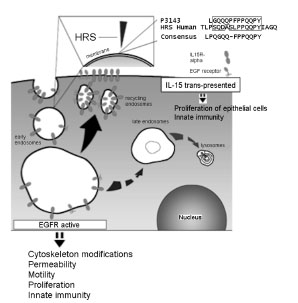
Figure 2. Overview of the P31-43 effects on the endocytic pathway. Due to a
sequence similarity with HRS, P31-43 interfered with its correct localization to the
endocytic membranes. The biological effects of this interference were: a) delayed
endocytic maturation, and b) increased recycling to the cell surface. P31-43-induced
delay of the active EGFR decay could activate several downstream signals with
different biological effects. The increase of the recycling pathway can direct more
transferrin receptor and probably other recycling receptors and IL-15 to the
membranes
Structural alterations
Different studies have identified the importance of the disruption of the integrity of the
epithelial layer in CD. One of the first structural alterations identified is the alteration
of the cell-to-cell junctional complexes that regulate intestinal permeability. Patients
show enhanced intestinal permeability and altered tight junction (TJ) morphology.
These disruptions persist in patients who are on a gluten-free diet (GFD) with a
normalized intestine, suggesting that permeability may play a driving role in the
development of CD. Moreover, epithelium integrity is impaired in the early stage of
the disease [3]. Polymorphisms in the TJ genes PARD3 and MAGI2 have been
associated with disease susceptibility in a Dutch cohort [3]. Interestingly, serine/
threonine-protein phosphatase 2A regulatory subunit B'' subunit alpha (PPP2R3A),
implicated in the negative control of cell growth, division and TJ regulation, remains
downregulated at the intestinal level in patients on a GFD. These observations suggest
a role for this pathway in the pathogenesis of CD [3].
Recent genetic studies point to the importance of polymorphisms of CD genes that are
involved in actin remodelling and cell adhesion. Among these, the lipoma-preferred
partner (LPP) gene presents the strongest non-HLA association signal, mapped in
intron 2 [3]. More recently, it has been suggested that deregulation of transcription
binding properties, due to single point mutations, might be the causal mechanism
underlying the association of CD with the LPP region [3]. The LPP protein localizes to
focal adhesions, which are the site of membrane attachment to the extracellular matrix
and cell-cell contact [3]. A constitutive alteration of LPP sub-cellular distribution
together with alterations of cell shape, actin cytoskeleton and focal adhesion has been
demonstrated in CD fibroblasts from GFD-patients [13]. Moreover, cell shape and
actin rearrangements are altered in CD dendritic cells from GFD-patients (data
submitted for publication).
Taken together, these data indicate that structural alterations are present in CD cells
independently of gluten.
Signalling and proliferation
Alterations in signalling pathways and cell proliferation have been demonstrated in
CD biopsies and cells (enterocytes, skin fibroblasts, dendritic/monocytes) of patients
on a gluten-free diet.
NF-B pathway
The nuclear factor 'kappa-light-chain-enhancer' of activated B-cells (NF-B) pathway
is constitutively altered in CD, with more than 20 components of the pathway
increased in GFD-CD biopsies. Most of the mRNA overexpressed in GFD-CD was
central to the regulation of the pathway [3]. Interestingly, two key mediators of the
NF-B pathway, Rel and TNFAIP3, have CD-associated gene variants [3]. It is widely
accepted that NF-B is a key regulator of inducible gene expression in the immune system. Both innate and adaptive immune responses, as well as the development and
maintenance of the cells and organs that comprise the immune system are, at multiple
stages, under the control of the NF-B family of transcription factors. Moreover, NF- B is responsible for the transcription of genes encoding a number of proinflammatory
cytokines and chemokines [3]. It has also been shown that NF-B is a
major mediator of IL-15 [3], which, among its many pleiotropic effects, is also able to
decrease claudin-2 levels in epithelial tight junction structures and leads to augmented
paracellular permeability, a phenomenon that is relevant and persistent in CD.
EGF receptor/ligand system
Constitutive activation of the EGF receptor/ligand system is also present in CD
enterocytes. Increases in EGFR protein levels, EGF mRNA, the downstream effector
molecule ERK and proliferation, which is ERK-dependent, have been found in
enterocytes from normal biopsies of GFD-CD patients [3].
Stress/innate immunity activation
Cellular stress
Cellular stress has been implicated in the early events of the disease, in particular in
the epithelium [3]. Heat shock protein-65 (HSP-65) is increased in CD enterocytes
before they develop the disease, indicating that epithelial stress may play a role in the
pathogenesis of CD. An alteration of this pathway was confirmed later with the
observation that HSP-72 increased [3]. More recently, a marker of ER cellular stress,
the molecular chaperone glucose-regulated protein 78 (Grp78), the master negative
regulator of the unfolded protein response (UPR), was found increased in enterocytes
with moderate and severe enteropathy and after at least two years on a GFD.
Remarkably, the increased peri-nuclear Grp78 aggregates co-localize with increased
major histocompatibility complex class I-related chain B (MICA/B+) in CD
enterocytes, linking cellular stress and innate immunity in CD.
IL-15/IL-15R alpha
IL-15 is elevated in intestinal biopsies from CD patients on a GFD [13]. In dendritic
cells from CD patients at all stages of the disease, more IL-15 has been found in the
cell membranes, indicating that this key mediator of the immune response is
constitutively altered in CD (Zanzi et al., manuscript submitted). In CD patients on a
GFD, the IL-15R alpha receptor has been observed at higher levels in intestinal
biopsies [4].
In conclusion, the data in the literature point to several constitutive alterations of cell
structure, signalling, proliferation and stress/innate immunity in CD cells. These
pathways, already constitutively altered in coeliac cells, render them more susceptible
to the effects of the gliadin peptides that can act on the same pathways. These same three sets of metabolic pathways can be triggered in normal cells by P31-43, which
mimics the coeliac cellular phenotype in controls.
Conclusion
In this short review we have shown most of the effects of gliadin peptide P31-43 in
intestinal biopsies and cells from normal subjects and CD patients at different stages of
the disease. We have highlighted the effects of an alimentary peptide, contained in
very common foods, that is biologically active on cell structure, signalling/proliferation
pathways and stress/innate immune activation. These are also constitutively
altered in coeliac cells and biopsies, rendering them more sensitive to the effects of
gliadin.
What remains to be understood is the molecular defect explaining the alterations of the
coeliac cells, most likely due to a particular genetic make-up. It is possible to
hypothesize that coeliac subjects have constitutive alterations that amplify the signals
induced by gliadin peptides.
Gluten itself could play a role in the pathogenesis of diseases different from CD, such
as type 1 diabetes. In children with insulin-dependent diabetes but not coeliac disease,
intestinal inflammation is triggered by viral infections and alimentary proteins [3]. In
particular, signs of an altered mucosal immune response to gliadin have been
described in type 1 diabetes both by challenging the rectum with gliadin peptides in
vivo [3], and the proximal small intestine in vitro [3]. In conclusion, gliadin and its
undigested peptides have biological effects not only in cells and the intestinal mucosa
of patients with CD but also in normal subjects or in different diseases. How these
effects can affect the health of non-coeliac subjects will be the object of future
research.
References
1. Veldhoen M, Brucklacher-Waldert V. Dietary influences on intestinal immunity.
Nat Rev Immunol 2012; 12: 696-708.
2. Marsh MN, Loft DE, Garner VG. Time dose responses of coeliac mucosae to
graded oral challenges with Frazer’s fraction III(Ff3) of gliadin. Europ
Gastroenterol Hepatol 1992; 4: 667-74.
3. Barone MV, Troncone R, Auricchio S. Gliadin peptides as triggers of the
proliferative and stress/innate immune response of the celiac small intestinal
mucosa. Int J Mol Sci 2015; 15: 20518-37. Review.
4. Sollid LM. Molecular basis of celiac disease. Annu Rev Immunol 2000; 18: 53-81.
5. Barone MV, Gimigliano A, Castoria G, et al. Growth factor-like activity of
gliadin, an alimentary protein: Implications for coeliac disease. Gut 2007; 56: 480-
8.
6. Juuti-Uusitalo K, Maki M, Kainulainen H, et al. Gluten affects epithelial
differentiation-associated genes in small intestinal mucosa of coeliac patients. Clin
Exp Immunol 2007; 150: 294-305.
7. Clemente MG, De Virgiliis S, Kang JS, et al. Early effects of gliadin on
enterocytes intracellular signalling involved in intestinal barrier function. Gut
2003; 52: 218-23.
8. Nanayakkara M, Lania G, Maglio M, et al. An undigested gliadin peptide activates
innate immunity and proliferative signaling in enterocytes: The role in celiac
disease. Am J Clin Nutr 2013; 98: 1123-35.
9. Caputo I, Secondo A, Lepretti M, et al. Gliadin peptides induce tissue
transglutaminase activation and ER-stress through Ca2+ mobilization in Caco-2
cells. PLoS One 2012; 7: e45209.
10. Di Sabatino A, Ciccocioppo R, Cupelli F, et al. Epithelium derived interleukin 15
regulates intraepithelial lymphocyte Th1 cytokine production, cytotoxicity, and
survival in coeliac disease. Gut 2006; 55: 469-77.
11. Barone MV, Nanayakkara M, Paolella G, et al. Gliadin peptide P31-43 localises to
endocytic vesicles and interferes with their maturation. PLoS One 2010; 5:
e12246.
12. Barone MV, Zanzi D, Maglio M, et al. Gliadin-mediated proliferation and innate
immune activation in celiac disease are due to alterations in vesicular trafficking.
PLoS One 2011; 6: e17039.
13. Nanayakkara M, Kosova R, Lania G, et al. A celiac cellular phenotype, with
altered LPP sub-cellular distribution, is inducible in controls by the toxic gliadin
peptide P31-43. PLoS One 2013; 8: e79763.
6.2 Consumption of wheat alpha-amylase trypsin inhibitors
(ATIs) accelerates murine systemic lupus
erythematosus
Victor Zevallos1, Julia Weinmann-Menke2, Myriam Meineck2, Detlef Schuppan1,3
1 Institute for Translational Immunology and Research Center for Immunotherapy
(FZI), Univ. Medical Center, Johannes Gutenberg University Mainz, Mainz,
Germany
2 Department of Nephrology and Rheumatology, Johannes-Gutenberg University
Mainz, Germany
3 Division of Gastroenterology, Beth Israel Deaconess Medical Center, Harvard
Medical School, Boston, USA
Introduction
Systemic lupus erythematosus (SLE) is an autoimmune disorder characterized by
severe and persistent inflammation that leads to tissue damage in multiple organs and
pathogenic autoantibodies. Kidneys are particularly affected leading to nephritis and
renal failure [1]. Although both genetic and environmental factors play an important
role in the disease mechanism, the aetiology remains unclear. However, the role of diet
as modulator of autoimmune diseases could help to explain this complex disease
mechanism.
We have identified wheat (rye, barley) alpha-amylase/trypsin inhibitors (ATIs) as
main nutritional triggers of innate immunity via the toll-like receptor 4 (TLR4)-MD2-
CD14 complex in cells of the mononuclear phagocyte system including monocytes
and dendritic cells [2]. ATIs are a family of non-gluten wheat proteins that represent
2-4% of the wheat protein and that are significantly present as “contaminating protein” in gluten and gliadin preparations [3]. Importantly, ATIs likely serve as major triggers
of non-celiac, non-allergy wheat sensitivity, which we characterize as worsening of a
pre-existent, mostly extraintestinal disease with the consumption of gluten- (and
therefore ATIs-) containing grains, flours and processed products [3-6].
The MRL-Fas(lpr) mouse develops progressive and spontaneous glomerular,
tubulointerstitial and perivascular kidney disease, arthritis, lymphadenopathy and
splenomegaly that replicates SLE. Therefore, we examined the effects of gluten-free
and gluten- (plus ATI-) containing diets on disease progression using the MRLFas(
lpr) mouse model.
Materials and methods
MRL-Fas(lpr) mice were placed either on a gluten-free (GFD/ATI-free) diet or a diet
containing 25% gluten (containing amounts of ATIs equivalent to the human wheat -based diet) for at least four weeks. We analysed clinical parameters such as
proteinuria, haematuria, haemoglobinuria and serum inflammatory
chemokine/cytokine levels throughout the experiment. At sacrifice, myeloid
inflammatory cells were quantified immunohistochemically in the intestine, kidneys
and spleen.
Results and discussion
Mice on a GFD showed significantly attenuated clinical parameters of kidney
dysfunction (proteinuria, haematuria, haemoglobinuria) and serum inflammatory
cytokines (IL-6, KC and TNFα) compared to mice on a gluten- (and ATI-) containing
diet. Gluten/ATI-fed mice also showed a significant increase in kidney infiltrating
CD4+ T cells producing IL-12 and IL-17. Both clinical and immunological findings
were in line with a worsened course in patients with SLE. Intestinal CD68+ and F4/80+
myeloid cells and CD4+ T cells were mildly increased in the intestine of mice
ingesting ATIs.
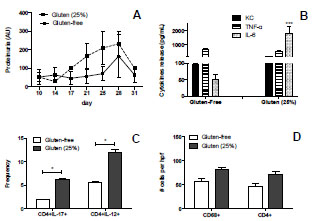
Figure 1. Evaluation of MRL-Fas(lpr) mice on a gluten-free compared to a gluten-
(and ATI-) containing diet. (A) Clinical score (proteinuria) during the experiment.
(B) Markers of inflammation (KC, TNF-α, and IL-6) in serum. (C) Isolated single cells
from mice’s kidneys, labelled with antibodies CD4 plus IL-17 and IL-12 and subjected
to FACS sorting. (D) Immunohistological number of intestinal CD68+ and CD4+ cells.
Level of significance (*p<0.05;***p<0.001) is represented by asterisks
Conclusion
We observed that dietary wheat ATIs (here combined with gluten), exacerbate the
development of SLE in mice. This effect is mediated by intestinal innate and
(secondary) adaptive immune activation. The gluten-free (and thus ATI-free) diet
seems to have a protective effect on the development of SLE in MRL-Fas(lpr) mice,
confirming the role of ATIs as major trigger of inflammation in autoimmune diseases.
Further studies need to dissect how the intestinal immune activation by ATIs
exacerbates the extra-intestinal manifestations of SLE.
References
1. Menke J, Rabacal WA, Byrne KT, et al. Circulating CSF-1 promotes monocyte
and macrophage phenotypes that enhance lupus nephritis. J Am Soc Nephrol 2009;
20: 2581-2592.
2. Schuppan D, Pickert G, Ashfaq-Khan M, et al. Non-celiac wheat sensitivity:
differential diagnosis, triggers and implications. Best Pract Res Clin Gastroenterol
2015; 29: 469-476.
3. Junker Y, Zeissig S, Kim S-J, et al. Wheat amylase trypsin inhibitors drive
intestinal inflammation via activation of toll-like receptor 4. J Exp Med 2012; 209:
2395-408.
4. Fasano A, Sapone A, Zevallos V, et al. Nonceliac gluten sensitivity.
Gastroenterology 2015; 148: 1195-1204.
5. Catassi C, Elli L, Bonaz B, et al. Diagnosis of non-celiac gluten sensitivity
(NCGS): The salerno experts’ criteria. Nutrients 2015; 7: 4966-4977.
6. Schuppan D, Zevallos V: Wheat amylase trypsin inhibitors as nutritional activators
of innate immunity. Dig Dis 2015; 33: 260-263.
7 Statements by participating organisations,
representatives from industry and guests
7.1 News from Codex and regulatory affairs
Hertha Deutsch
AOECS Codex Delegate, Austrian Coeliac Society, Vienna
Introduction
AOECS, the Association of European Coeliac Societies, has Observer status in the
Codex Alimentarius Commission since 1992. Information about the organisation, the
duties and all the extensive work of all Codex Committees is published on their
website [1]. From November 2014 till July 2015 three important Codex sessions took
place, where issues regarding “gluten-free” were discussed and adopted: Codex
Committee on Nutrition and Foods for Special Dietary Uses (CCNFSDU) [2], Codex
Committee on Methods of Analysis and Sampling (CCMAS) [3] and Codex
Alimentarius Commission (CAC) [4]. Furthermore, on 13 December 2014 the EU
Regulation 1169/2011 came into force with further improvements for coeliacs [5].
CCNFSDU
The thirty-sixth session of the CCNFSDU took place from 24 - 28 November 2014 in
Bali, Indonesia, [2] and was attended by 299 delegates representing 54 Member
Countries, one Member Organisation and 25 International Organisations, one of them
being the AOECS. At the adoption of the agenda the Committee agreed to discuss
among other items under agenda item 11 “Other Business” also the proposals of
Austria and USA:
“Proposal for an extension of the method recommendation in the Standard
for Foods for Special Dietary Use for Persons Intolerant to Gluten
(CODEX STAN 118-1979) with a method that also accurately detects the
toxic fraction in gluten harmful for individuals intolerant to gluten: the
ELISA G12 method” (Austria)
“Proposal to amend the Standard for Foods for Special Dietary Use for
Persons Intolerant to Gluten (CODEX STAN 118-1979) to add the term “khorasan wheat” (USA)
G 12 method
At agenda item 11, Austria presented the proposal and recommended that since the
ELISA G12 method fulfilled all the criteria stated in 5.1 in the CODEX STAN 118-
1979, and was supported by inter-laboratory validation data and internationalapprovals, it should be incorporated into CODEX STAN 118-1979 and therefore
proposed to refer the proposal to CCMAS for consideration.
On behalf of AOECS, I recalled that the threshold for “gluten-free” as defined in
CODEX STAN 118-1979 was determined by analysing foods with the R5 method.
Therefore, I requested that before taking a decision, consideration should be made
regarding the labelling consequences for the term “gluten-free” before incorporating
the G12 method into the standard, should the results determined by the G12 in the
same food samples be different than those determined by the R5 method.
Conclusion - G 12 method
The Committee agreed to ask CCMAS to examine ELISA G12 as a potential
additional method.
Kamut
Already at the session of the CCFSDU in November 2013 [6] the representative of
Belgium suggested to replace the term “kamut” with “khorasan wheat” in the Standard
for Foods for Special Dietary Use for Persons Intolerant to Gluten.
On behalf of AOECS, I reported that “kamut” is a widely used cereal name well
known to consumers, food producers and all Codex member states and requested not
to remove this name from the list of gluten-containing cereals as it would be
understood that kamut does not contain gluten and this would cause very severe and
adverse consequences for the health of the gluten-intolerant population worldwide.
In the Conference Room Document (CRD) 26 for the CCNFSDU session of
November 2014 [1,2] the delegation of USA acknowledged the concern of AOECS
and in order to facilitate consensus, USA proposed to amend the wording: “and
khorasan wheat, which is marketed under the trademarks such as KAMUT”.
Conclusion - kamut
The Committee modified the proposed wording and agreed to forward the amendment
to CODEX STAN 118-1979 to CAC 38 for adoption:
AMENDMENTS TO THE CODEX STANDARD FOR FOODS FOR
SPECIAL DIETARY USE FOR PERSONS INTOLERANT TO
GLUTEN (CODEX STAN 118-1979)
2.1.1 Gluten-free foods
Gluten-free foods are dietary foods
a) consisting of or made only from one or more ingredients which do not
contain wheat (i.e., all Triticum species, such as durum wheat, spelt, and
khorasan wheat, which is also marketed under different trademarks such
as KAMUT), rye, barley, oats* or their crossbred varieties, and the glutenlevel does not exceed 20 mg/kg in total, based on the food as sold or
distributed to the consumer, and/or
b) consisting of one or more ingredients from wheat (i.e., all Triticum
species, such as durum wheat, spelt, and khorasan wheat, which is also
marketed under different trademarks such as KAMUT) rye, barley, oats*
or their crossbred varieties, which have been specially processed to remove
gluten, and the gluten level does not exceed 20 mg/kg in total, based on the
food as sold or distributed to the consumer.
2.1.2 Foods specially processed to reduce gluten content to a level above
20 up to 100 mg/kg
These foods consist of one or more ingredients from wheat (i.e., all
Triticum species, such as durum wheat, spelt, and khorasan wheat, which
is also marketed under different trademarks such as KAMUT), rye,
barley, oats* or their crossbred varieties, which have been specially
processed to reduce the gluten content to a level above 20 up to 100 mg/kg
in total, based on the food as sold or distributed to the consumer.
The Codex secretary explained that usually it is not possible to have a trademark name
in a Codex Standard, however, because this issue is strongly related to a health hazard,
the legal adviser of Codex approved this exception.
CCMAS
The thirty-sixth session of the CCMAS took place from 23 - 27 February 2015 in
Budapest, Hungary [3] and was attended by 52 member countries, one member
organisation and observers from 11 international organisations, one of them being the
AOECS.
A few days before the CCMAS session, the Inter-Agency Meeting (IAM) took place.
The report is published in CRD 3 [1, 3]. Regarding methods for gluten determination,
their conclusion was:
“The participants discussed the status of the R5 and G12 methods for
gluten determination. AACCI reported that two separate collaborative
trials had been carried out successfully with R5 and G12 on maize- and
rice-containing foods, respectively. AACCI has approved each method with
scope restricted to the matrices used in the collaborative trials. AACCI
proposes to remove the “all food” designation for R5 methods and include
G12. In essence both methods had recently been fully validated by
collaborative trial and are published by AACCI as:
R5 method: AACC Intl 38-50.01 (immunoassay procedure (validated using
maize matrices)) and G12 method: AACC Intl 38-52.01 (immunoassay
procedure (validated using rice matrices))”
The Codex Working Group (WG) on the endorsement of methods of analysis and
sampling was held on Saturday, 21 February 2015, prior to the plenary session.
Among other items, the WG discussed also the method for detection of the toxic
fraction in gluten harmful for individuals, which was one of some subjects on agenda
item 2, published in document CX/FH 15/36/2 [3]. The delegate of AACCI repeated
their suggestion as written above.
On behalf of AOECS, I did not agree to delete “all foods” because coeliacs need a
reliable method for all various kinds of foods in the CODEX STAN 118-1979. To
focus on maize matrices and rice matrices is not really helpful in practice because
there are a large variety of gluten-free ingredients/foods on the market and the
question appears how to deal with potential different analytical results of the same
food sample if both methods will be classified as Type 1 method. For the time being
we do not have enough data to be sure that both methods show the same results in
various food samples. Further on, it is well known that the G12 antibody has a weak
cross-reactivity to some oats samples and it needs to be investigated whether the
positive signal comes from contamination with wheat, rye or barley or from a specific
oats cultivar which cross-reacts with the G12 antibody.
The conclusion of the consideration of the WG on the endorsement of methods of
analysis and sampling is published in CRD 2 [1,3]:
“Method for detection of the toxic fraction in gluten harmful for individuals
The WG noted that it was not possible to have two Type I methods and that
if ELISA G12 method were to be added, the provision in the Standard
would need to be differentiated to allow for both methods to be included as
Type I methods. The WG noted that ELISA G12 had been validated for
gluten-free foods, rice matrices, whereas R5 had been validated for glutenfree
foods, maize matrices. Both methods had recently been fully validated
by collaborative trial and are published by AACCI as:
R5 method: AACC Intl 38-50.01 (immunoassay procedure (validated using
maize matrices)) and
G12 method: AACC Intl 38-52.01 (immunoassay procedure (validated
using rice matrices))
The WG recommended that decision in this regard should be taken by
CCNFSDU.”
In the plenary session during the consideration of this item the comments were the
same as in the WG and few other delegations participated in the discussion, but did not
have other proposals or suggestions for a solution. Usually the recommendation of the
WG is adopted in the plenary session and this was also the case regarding this item.
Conclusion - CCMAS
CCMAS recommended that decision in this regard should be taken by CCNFSDU.
Further items on the agenda important for coeliacs were the consideration of a
discussion paper on the development of procedures/guidelines for determining
equivalency to Type 1 methods. This paper had not been completed in time to allow
for discussion in the electronic WG space, it was presented as a first draft and work
will continue.
At the agenda the item “Review and Update of Methods” the R5 was not part of the
work package, however, work will go on to review all existing methods.
CAC
The thirty-eight session of the CAC took place from 6 - 11 July 2015 in Geneva,
Switzerland [4] and was attended by delegates from 140 Member Countries, one
Member Organisation, 33 International Governmental and Non-Governmental
Organisations, including UN agencies and AOECS.
The most important task of the Codex Commission is to adopt Codex Standards and
Guidelines which are usually taken into national legislation by governments. Also the
World Trade Organisation refers to Codex Standards in matters of import, export and
legal issues. In the Commission session, also decisions were taken about the budget,
the Trust Fund (= financial support for delegates from developing countries to
participate in Codex sessions and to implement Codex Standards in their countries),
adoption of new work or to revoke existing Standards.
The most important issue on the agenda for coeliacs was item 5 (f) “Amendment to
Codex Standards and Related Texts” which concerned the above written amendments
to CODEX STAN 118-1979 - see under “Conclusion - Kamut” of this paper.
Conclusion - CAC
The Codex Commission adopted the amendments without any further discussion.
FAO GM Food Platform
During lunch break also “side events” took place. The most important session was the
FAO GM Food Platform, which is an online platform to share information on the
safety assessment of foods derived from recombinant DNA plants authorized in
accordance with the “Guideline for the conduct of food safety assessment of foods
derived from recombinant DNA plants”. The FAO GM Foods Platform is open to the
public. As of 18 June 2015, a total of 164 members have nominated focal points for
the Platform. 105 members have completed their country profile and the Platform
hosts 733 national records of the results of GM food safety assessment. After some
presentations delegates could make some comments or ask questions.
Also this year, I recalled § 42 and 43 of the “Guideline for the conduct of food safety
assessment of foods derived from recombinant DNA plants”, informed about the
incidence/prevalence of CD and gluten sensitivity and asked if there is any controlmechanism installed that the text of the Guideline not to transfer genes from glutencontaining
cereals to foods gluten-free by nature is really considered in practice. Only
the delegate of Australia answered that he was aware of this issue.
Conclusion - FAO GM Food Platform
How is this very imported issue monitored by the rest of the 163 members of the FAO
GMO Food Platform?
EU Regulation 1169/2011
This Regulation [5] on the provision of food information to consumers comprises
several Regulations and Directives of previous years. The labelling of gluten
containing substances or products in pre-packed foods remained unchanged and is
published in Annex II. Some new and further improvements for consumers in terms of
general labelling information are published, the most important is written in Article
44: National measures for non-prepacked food. This very important item came into
force on 13 December 2014 and enables any consumer suffering either from allergy or
intolerance to obtain correct information also e.g., in any restaurant in the EU which
uses a substance from Annex II in a food. The first item in this Annex II is “cereals
containing gluten and products thereof”.
Conclusions
As in the past, also this year some benefits regarding food regulatory improvements
for coeliacs were achieved. However, some important items are still open for
discussion, consideration and further work.
References
1. Codex Alimentarius Commission www.codexalimentarius.org/.
2. Report of the thirty-sixth session of the Codex Committee on Nutrition and Foods
for Special Dietary Uses, Bali, Indonesia, 24 - 28 November 2014.
3. Report of the thirty-sixth session of the Codex Committee on Methods of Analysis
and Sampling, Budapest, Hungary 23 - 27 February 2015.
4. Joint FAO/WHO Standards Programme, Codex Alimentarius Commission, thirtyeight
session, CICG, Geneva, Switzerland, 6 - 11 July 2015.
5. Regulation (EU) No 1169/2011 of the European Parliament and of the Council of
25 October 2011 on the provision of food information to consumers.
6. Report of the thirty-fifth session of the Codex Committee on Nutrition and Foods
for Special Dietary Uses, Bad Soden am Taurus, Germany, 4 - 8 November 2013.
7.2 Update on activities CEN/TC 275/WG 12 "Food
allergens"
Rupert Hochegger
Austrian Agency for Health and Food Safety (AGES), Vienna, Austria
Abstract
Molecular and immunological analytical methods are currently the recognized tools
for determination of DNA and protein analytes in foods.
Sampling and analysis methods used in the context of official controls shall comply
with relevant Community rules or, if no such rules exist, with internationally
recognized rules or protocols, for example those that the European Committee for
Standardization (CEN) has accepted or those agreed in national legislation.
One of the goals of CEN technical committee 275 “food analysis” is to support the
harmonization of molecular and immunological methods for detection, identification
and quantification of specific DNA sequences and specific proteins in foods, which
produce comparable results when performed at different laboratories.
The scope of working group (WG) 12 - food allergens - is to standardize methods for
the detection and determination of potentially allergenic substances in foodstuffs or
markers for their presence, including but not limited to immunological and molecularbiological
methods.
At the last WG meeting it was agreed to draft a document for the definition of
minimum performance requirements for ELISA methods. The standard document shall
be allergen-specific and define analytical performance criteria of the respective
method. The scope of this document is to provide recommendations on how ELISA
methods for food allergen analysis shall be evaluated and validated in order to ensure
that comparable and reproducible results are obtained in different laboratories.
To be widely used by official control laboratories, there is synergy between the
recommendations that will be made within this document and those of the Codex
Alimentarius Commission.
8 Perspectives and action plan of the PWG
Peter Koehler
Deutsche Forschungsanstalt für Lebensmittelchemie, Leibniz Institut, Freising, Germany
The Prolamin Working Group executive meeting and joint discussion held on
9 October 2015, led to the decisions and statements outlined below.
Action plan
I. Analytical
Peter Koehler is responsible for the PWG gliadin reference material
(peter.koehler@tum.de).
PWG gliadin will continue to be the reference material supported by the group.
Material for 5 - 10 years is still on stock.
The group will wait for the first MoniQA reference flours and will then decide
how to proceed with novel extracted reference material(s). No additional
reference will be prepared before, because a suitable reference material is
available.
An increase of the price was discussed, but without final decision.
II. Clinical
For the symposium of the 2016 meeting the topic “Enzymatic gluten
degradation” has been selected. Speakers will be Andreas Frey and Eva
Helmerhorst.
A speaker on innate lymphoid cells or the importance of the microbiome in
gluten intolerances will be invited.
III. Members, Policy
Olivier Tranquet and Rudolf Valenta have been asked to join the group and
have accepted.
A joint EU research project (Horizon 2020) could deal with all “Wheat
hypersensitivities”. A suitable call is looked for or will be generated (Troncone,
Schuppan, Gilissen, Koning, Koehler).
The website will be further improved (Chirdo, Koehler).
This printed, citable book (print run: 300 copies with ISBN number) was made
possible by funding of Dr. SCHÄR GmbH/Srl, (Burgstall, BZ, Italy) and by the
help of Mrs. Anneliese Stoiber and Dr. Gaby Andersen, Deutsche Forschungsanstalt
für Lebensmittelchemie (Freising, Germany). It will be distributed
among leaders of opinion in gluten analysis and clinical medicine. An
electronic version can be downloaded from the PWG website.
Next meeting: 2016
We are very pleased to announce the venue for our meeting in 2016:
Valencia, Spain
Host:
Prof. Dr. Cristina M. Rosell
Institute of Agrochemistry and Food Technology (IATA-CSIC)
E-mail: crosell@iata.csic.es
Time: 22 - 24 September 2016
Focus of the meeting:
Enzymatic gluten degradation
Gluten quantitation (immunochemical/non-immunochemical)
Gluten reference materials for analytical and clinical studies
The meeting will be limited to 55 participants and attendance is by
invitation only. Invitations have been sent by March 2016. Registration
deadline will be June 15, 2016.
For registration please contact:
Maria Saneustaquio
Institute of Agrochemistry and Food Technology (IATA-CSIC)
Avenida Agustin Escardino, 7
46980 Paterna, Valencia, Spain
Phone: +34 963 900022
Fax: +34 963 636301
E-mail: marivise@iata.csic.es
Very special thanks to the host of this kind invitation!
|
|
List of Participants
GROUP MEMBERS
Prof. Dr. Fernando G. Chirdo
Laboratorio de Investigación en el Sistema Inmune (LISIN)
Facultad de Ciencias Exactas Universidad Nacional de La Plata cc 711
(1900) LA PLATA, ARGENTINA
Phone: +54 221 421 0 497 | 423 0 121 | 423 5 333 (Int 45)
Tele fax +54 221 422 6947
Email: fchirdo@biol.unlp.edu.ar
Prof. Paul J. Ciclitira
King's College London (Division of Diabetes and Nutritional Sciences) The Rayne Institute (KCL). St Thomas' Hospital Westminster Bridge Road. LONDON SE1 7EH, UK/ENGLAND
Phone: +44 207 620 2597 | 207 188 2494 | Telefax +44 207 261 0667
Email: mila.labar_weintrop@kcl.ac.uk (secretary)
Email: paul.ciclitira@kcl.ac.uk
Prof. Conleth Feighery, MD
(not at tend ing)
University of Dublin Department of Immunology St. James's Hospital James's Street DUB LIN 8, IRELAND
Phone: +353 1 896 3432 | Tele fax +353 1 4545-609
Email: con.feighery@tcd.ie
Dr. Luud Gilissen
Plant Research International (PRI) Wageningen University
Droevendaalsesteeg 1 6708 PB WAGENINGEN, THE NETHERLANDS
Phone: +31 317-480983 | Fax: +31 317-418094
Email: luud.gilissen@wur.nl
Prof. Dr. Peter Köehler
Deut sche Forschungsanstalt für Lebensmittelchemie
Lise-Meitner-Straße : +34 - 85354 FREISING, GER MANY
Phone: +49 81 61 71 29 28 | Tele fax +49 81 61 71 29 70
Email: peter.koehler@tum.de
Prof. Dr. Frits Koning
Leiden Univer sity Medical Center, E3-Q
Department of Immunohaematology and Bloodbank Albinusdreef 2
2333 ZA LEIDEN, THE NETHERLANDS
Phone: +31 71 5266673 | Tele fax +31 71 5265267
Email: fkoning@lumc.nl
Prof. Dr. Thomas Mothes
Universitätsklinikum Leip zig A. ö. R.
Institut für Laboratoriumsmedizin, Klinische Chemie und Molekulare Diagnostik - Liebigstraße : 27 - 04103 LEIPZIG, GERMANY
Phone: +49 341 97 22251 | Tele fax +49 341 97 22329
Email: mothes@medizin.uni-leipzig.de
Prof. Dr. Dr. Detlef Schuppan
I. Medizinische Klinik und Poliklinik Universitätsmedizin der Johannes
Gutenberg-Universität Mainz Langenbeckstr. 1,55131 MAINZ, GERMANY
Phone +49 6131-177355/ 177356/177104 - Fax +49 6131-177357
Email: detlef.schuppan@unimedizinmainz.de
Prof. Dr. Riccardo Troncone (not attending)
Department of Pediatrics and European Laboratory for the Investigation of Food-induced Diseases University of Naples “Federico II”- via Pansini, 5 - 80131 NAPLES, ITALY
Phone: +39 081 7463383 | Telefax +39 081 5469811
Email: troncone@unina.it
HOSTS
Mag. Simone Schreiter
Romer Labs Division Holding GmbH
Erber Campus 1
3131 GETZERSDORF, AUSTRIA
Phone: +43 2782 803 11608
E-mail: simone.schreiter@romerlabs.com
INVITED SPEAKER
Dr. Valentina Discepolo
Post-Doctoral Scholar
Department of Medicine
University of Chicago
900 E 57th Street
60615 CHICAGO (IL), USA
Phone (It): +39 338 3709881
Phone (USA): +1 312 7742790
E-mail: vale.discepolo@gmail.com
Prof. Dr. Roland Ernest Poms
MoniQA Association
Marxergasse 2
1030 VIENNA, AUSTRIA
Phone: +43 1 7077202 0
Fax: +43 1 7077204 0
E-mail: roland.poms@moniqa.org
GUESTS
Mr. Dave Almy
Neogen Corporation
620 Lesher Place
48912 LANSING, USA
Phone: +1 517 372 9200
Fax: +1 517 367 0514
E-mail: dalmy@neogen.com
Mrs. Tova Almlöf
Semper AB
Semper AB Box 1101
SE 17222 SUNDBYBERG, SWEDEN
Phone : +46 767232862
E-mail: tova.almlof@semper.se
Mrs. Sofia Beisel
Deutsche Zöliakiegesellschaft e.V.
Kupferstr 36
70565 STUTTGART, GERMANY
Phone: +49 711 45998115
Fax: 49 711 459981 50
E-mail: sofia.beisel@dzg-online.de
Dr. Markus Brandt
Ernst Böcker GmbH & Co KG
Ringstrasse 55-57
32427 MINDEN, GERMANY
Phone: +49 571 837990
Fax: +49 571 8379920
E-mail: markus.brandt@sauerteig.de
Dr. Kurt Brunner
Romer Labs Division Holding GmbH
Technopark 1
3430 TULLN, AUSTRIA
Phone: +43 2272 615 33 0
E-mail: kurt.brunner@romerlabs.com
Dr. Virna Cerne
Dr. Schär AG /SPA
Winkelau 9
39014 BURGSTALL, ITALY
Phone: +39 0403755380
Fax: +39 0403755385
E-mail: virna.cerne@drschaer.com
Dr. Michelle Colgrave
CSIRO
306 Carmody Rd, St Lucia
4067 BRISBANE, AUSTRALIA
Phone: +61 42 2670005
Fax: +61 732142900
E-mail: michelle.colgrave@csiro.au
Mr. Henrik Dahlquist
Fria Gluten Free
Fältspatsgatan 12
43021 VÄSTRA FRÖLUNDA,
SWEDEN
Phone: +46 70 654 600
E-mail: henrik.dahlquist@fria.se
Dr. Johan De Meester
Cargill R&D Centre Europe
Havenstraat 84
B-1800 VILVOORDE, BELGIUM
Phone: +32 473997653
E-mail: Johan_De_Meester@cargill.com
Mrs. Hertha Deutsch
Österreichische Arbeitsgemeinschaft
Zöliakie
Anton Baumgartner Straße 44/C5/2302
1230 VIENNA, AUSTRIA
Phone: +43 166 71887
E-mail: hertha.deutsch@chello.at
Dr. Clyde Don
CDC FoodPhysica
Vogelwikke 12
6665 HP DRIEL, THE NETHERLANDS
Phone: +31 622 543047
E-mail: clyde.don@foodphysica.com
DI Mag. Julia Edlmair
Austrian Coeliac Society
Lederergasse 27
4020 LINZ, AUSTRIA
Phone: +43 650 9913557
E-mail: glutenfrei@zoeliakie.or.at
Mr. Richard Fielder
Bio-Check (UK)
Spectrum House, Llys Edmund Prys,
St. Asaph Business Park
LL17 0JA ST. ASAPH, UK
Phone: +44 1745 335165
Fax: +44 1745 582867
E-mail: richard@biocheck.uk.com
Mr. Phil Goodwin
Bio-Check (UK)
Spectrum House, Llys Edmund Prys,
St. Asaph Business Park
LL17 0JA ST. ASAPH, UK
Phone: +44 1745 335165
Fax: +44 1745 582867
E-mail: phil@biocheck.uk.com
Dr. Thomas Grace
Bia Diagnostics
480 Hercules Dr.
5446 COLCHESTER, VT, USA
Phone: +1 802 540 0148
E-mail: thomasgrace@biadiagnostics.
Mrs. Robin Grace
Bia Diagnostics
480 Hercules Dr.
5446 COLCHESTER, VT, USA
Phone: +1 802 540 0148
E-mail: robin@biadiagnostics.com Mrs. Gertrud Granel
Association of German Cereal
Processors and Starch Producers
(VDGS e.V.)
Johannesstraße 37
53225 BONN, GERMANY
Phone: +49 30 8892368915
Fax: +49 30 88923689 19
E-mail: granel@vdgs.org
Dr. Antonio Guillén
INGENASA
C/García Noblejas 39
28037 MADRID, SPAIN
Phone: +34 91 650643322
E-mail: aguillen@ingenasa.com
Dr. Sigrid Haas-Lauterbach
R-Biopharm AG
An der neuen Bergstrasse 17
64297 DARMSTADT, GERMANY
Phone: +49 6151 81 02 25
E-mail: s.h.lauterbach@r-biopharm.de
Ms. Katri Hautanen
Fria Gluten Free
Fältspatsgatan 12
421 30 VÄSTRA FRÖLUNDA,
SWEDEN
Phone: +46 70 5156683
E-mail: katri.hautanen@fria.se
Dr. Crispin Howitt
CSIRO - Agriculture Flagship
GPO Box 1600
2601 CANBERRA, AUSTRALIA
Phone: +61 26 2465032
E-mail: Crispin.Howitt@csiro.au
Mr. Xin Huang
University of Helsinki
Agnes Sjöbergin katu 2, PL66
14 HELSINKI, FINLAND
Phone: +358 451210203
E-mail: xin.huang@helsinki.fi
Dr. Ulrike Immer
R-Biopharm
An der neuen Bergstraße 17
64297 DARMSTADT, GERMANY
Phone: +49 6151 8102 186
Fax: +49 6151 8102 40
E-mail: u.immer@r-biopharm.de
Dr. Päivi Kanerva
Fazer Mill & Mixes
Kasakkamäentie 3
FI-15800 LAHTI, FINLAND
Phone: +358 40 6605117
E-mail: paivi.kanerva@fazer.com
Dr. Götz Kröner
Hermann Kröner GmbH
Lengericher Str. 158
49479 IBBENBÜREN, GERMANY
Phone: +49 5451 9447 11
Fax: +49 5451 9447 811
E-mail: kroener@kroener-staerke.de
Ms. Karoline Kröner
Hermann Kröner GmbH
Lengericher Str. 158
49479 IBBENBÜREN, GERMANY
E-mail: karoline.kroener@web.de
Mrs. Stelle Lindeke
R-Biopharm AG
An der neuen Bergstraße 17
64297 DARMSTADT, GERMANY
Phone: +49 6151 8102 92
E-mail: s.lindeke@r-biopharm.de
Ms. Kathryn Miller
Coeliac UK
3rd Floor, Apollo Centre, Desborough
Road
HP11 2QW HIGH WYCOMBE, UK
Phone: +44 01494796130
E-mail: kathryn.miller@coeliac.org.uk
Dr. Jonatan Miranda
University of the Basque Country
UPV/EHU
Paseo de la Universidad, 7
1006 VITORIA-GASTEIZ, SPAIN
Phone: + 34 945013069
Fax: + 34 945013014
E-mail: jonatan.miranda@ehu.es
Dr. Sandra Pahr
Division of Immunopathology,
Department of Pathophysiology
and Allergy
Währingergürtel 18-20, 3Q
1090 VIENNA, AUSTRIA
Phone: +43 4040051090
E-mail: sandra.pahr@meduniwien.ac.at
Mrs. Catherine Remillieux-Rast
Association Française des Intolérants
au Gluten (AFDIAG)
23 Rue de Venise
78740 VAUX-SUR-SEINE, FRANCE
Phone: +33 681270911
Fax: +33 130993668
E-mail: c.remillieux_rast@yahoo.fr
Dr. Cristina Romero
INGENASA
C/Hermanos García Noblejas, 39
28037 MADRID, SPAIN
Phone: +34 91 368 05 01
Fax: +34 91 408 75 98
E-mail: cromero@ingenasa.com
Prof. Christina Rosell
Institute of Agrochemistry and Food
Technology (IATA-CSIC)
Avenida Agustin Escardino, 7
46980 PATERNA, SPAIN
Phone: +34 963 900022
Fax: + 34 963 636301
E-mail: crosell@iata.csic.es
Mr. Nermin Sajic
EuroProxima B.V.
Beijerinckweg 18
6827 BN ARNHEM, THE
NETHERLANDS
Phone: +31 263630364
Fax: +31 263645111
E-mail: nermin.sajic@europroxima.com
Dr. Martin Salden
EuroProxima B.V.
Beijerinckweg 18
6827 BN ARNHEM, THE
NETHERLANDS
Phone: +31 26 3630364
Email: m.salden@noviosmart.eu
Mrs. Kathrin Schalk
Deutsche Forschungsanstalt für
Lebensmittelchemie
Lise-Meitner-Str. 34
85354 FREISING, GERMANY
Phone: +49 8161 712926
E-mail: kathrin.schalk @lrz.tum.de
Dr. Katharina Scherf
Deutsche Forschungsanstalt für
Lebensmittelchemie
Lise Meitner-Strasse 34
85354 FREISING, GERMANY
Phone: +49 8161712927
Fax: +49 8161712970
E-mail: katharina.scherf@lrz.tum.de
Dr. Juan Ignacio Serrano-Vela
Asociacion de Celiacos de Madrid
Calle Lanuza 19-bajo
28028 MADRID, SPAIN
Phone: +34 917130147
Fax: +34 917258059
E-mail:
nachoserrano@celiacosmadrid.org
Dr. Edurne Simón
University of the Basque Country
UPV/EHU
Paseo de la Universidad, 7
1006 VITORIA-GASTEIZ, SPAIN
Phone: + 34 945013069
Fax: + 34 945013014
E-mail: edurne.simon@ehu.es
Dr. Tuula Sontag-Strohm
University of Helsinki
Agnes Sjöbergin katu 1
00014, HELSINKI, FINLAND
Phone: +358 504487467
E-mail: tuula.sontag-strohm@helsinki.fi
Ylva Sjögren Bolin
Swedish Food Agency
P.O. Box 622
SE-751 26 UPPSALA, SWEDEN
Phone +46 18 171416
Telefax +46 18 105848
Email: ylva.sjogren@slv.se
Mrs. Pauline Titchener
Neogen Europe Ltd.
The Dairy School, Auchincruive
KA6 5HU AYR, SCOTLAND, UK
Phone: +44 1292 525 600
Fax: +44 1292 525 601
E-mail: p.titchener@neogeneurope.com
Dr. Sándor Tömösközi
BME
Műegyetem rkp. 3.
H-1111 BUDAPEST, HUNGARY
Phone: +36 14631419
Fax: +36 14633855
E-mail: tomoskozi@mail.bme.hu
Dr. Olivier Tranquet
INRA
Rue de la Géraudière BP 71627
44316 NANTES CEDEX 3, FRANCE
Phone: +33 2 40675027
Fax: +33 240675025
E-mail: olivier.tranquet@nantes.inra.fr
Dr. Angel Venteo
INGENASA
C/Hermanos García Noblejas nº 41
28037 MADRID, SPAIN
Phone: +34 91 3680501
Fax: +34 91 4087598
E-mail: aventeo@ingenasa.com
Dr. Eva Maria Wanzenböck
Romer Labs Division Holding GmbH
Erber Campus 1
3131 GETZERSDORF, AUSTRIA
Phone: +43 2782 803 0
Fax: +43 2272 61533 13177
E-mail: eva.wanzenboeck@romerlabs.com
Ms. Hellen Watson
Ghent University
Valentin Vaerwyckweg 1
9000 GENT, BELGIUM
Phone: +32 9 242 42 36
Fax: +32 9 242 42 79
E-mail: hellen.watson@ugent.be
Dr. Thomas Weiss
R-Biopharm AG
An der neuen Bergstrasse 17
64297 DARMSTADT, GERMANY
Phone: +49 6151 8102 186
Fax: +49 6151 8102 40
E-mail: t.weiss@r-biopharm.de Mrs. Maren Wiese
Hermann Kröner GmbH
Lengericher Straße 158
49479 IBBENBÜREN, GERMANY
Phone: +49 5451 9447 12
Fax: +49 5451 9447 812
E-mail: wiese@kroener-staerke.de
Ms. Nathalie Widmann
R-Biopharm
An der neuen Bergstraße 17
64297 DARMSTADT, GERMANY
Phone: +49 6151 8102 7220
Fax: +49 6151 8102 40
E-mail: N.Widmann@r-biopharm.de
Dr. Johannes Wolf
Universitätsklinikum Leipzig A.ö.R.
Institut für Laboratoriumsmedizin,
Klinische Chemie und Molekulare
Diagnostik
Liebigstraße 27
04103 LEIPZIG, GERMANY
Phone: +49 341 97 22454
Fax: +49 341 97 22373
E-mail: johannes.wolf@medizin.unileipzig.de
Dr. Victor Zevallos
I. Medizinische Klinik und Poliklinik
Universitätsmedizin der Johannes
Gutenberg-Universität Mainz
Institut für Translationale Medizin
Obere Zahlbacher Str. 63
55131 MAINZ, GERMANY
Phone: +49 6131 17 9783
Fax: +49 6131 17 9988
Email: zevallos@uni-mainz.de
Impressum
Proceedings of the 29th Meeting
WORKING GROUP
on PROLAMIN ANALYSIS and TOXICITY
8 - 10 October 2015
Tulln, Austria
This work including all parts is subject to copyright. All rights are reserved and any
utilisation is only permitted under the provisions of the German Copyright Law.
Permissions for use must always be obtained from the publisher. This is in particular
valid for reproduction, translation, conversion to microfilm and for storage or
processing in electronic systems.
Scientific Organisation
Prof. Dr. Peter Koehler
Deutsche Forschungsanstalt für Lebensmittelchemie
Lise-Meitner-Str. 34, 85354 FREISING, GERMANY
Phone: +49 8161 712928; Fax: +49 8161 712970
Email: peter.koehler@tum.de
Host
Mag. Simone Schreiter
Romer Labs Division Holding GmbH
Erber Campus 1, 3131 Getzersdorf, Austria
Phone: +43 2782 803 11608
E-mail: simone.schreiter@romerlabs.com
Cover picture* and picture of participants
Thomas Mothes
© Verlag Deutsche Forschungsanstalt für Lebensmittelchemie (DFA)
Lise-Meitner-Strasse 34, 85354 Freising
Phone: +49 8161 712928; Fax: +49 8161 712970
dfa@lrz.tum.de / www.dfal.de
ISBN: 978-3-946117-02-5 |